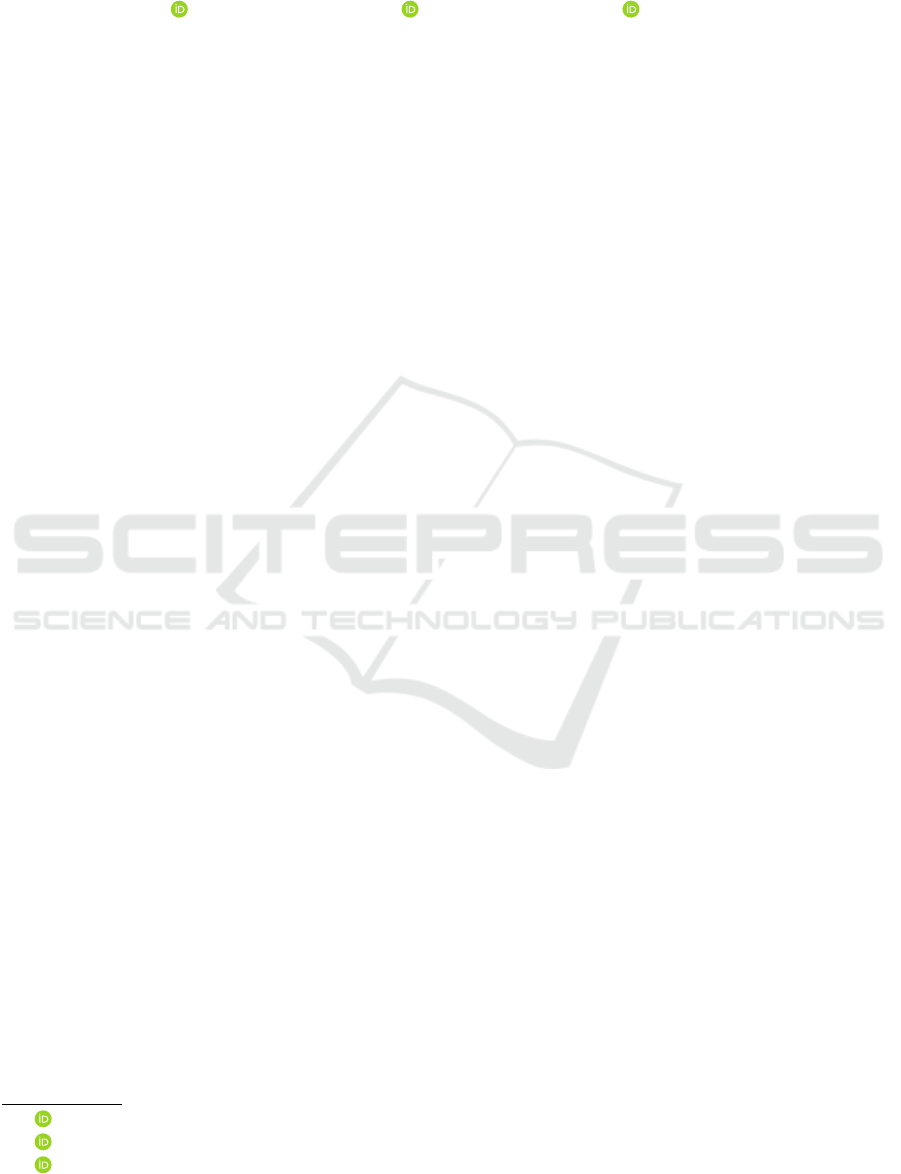
High Speed Measurement in Spectral Drill using Q-plate and Camera
Seigo Ohno
1 a
, Katsuhiko Miyamoto
2 b
, Shin’ichiro Hayashi
3 c
and Norihiko Sekine
3
1
Department of Physics, Tohoku University, 6-3, Aza-Aoba, Aoba-ku, Sendai, Japan
2
Department of Materials Science, Chiba University, 1-33, Yayoi-cho, Inage-ku, Chiba, Japan
3
Terahertz Technology Research Center, National Institute of Information and Communications Technology,
4-2-1 Nukui-Kitamachi, Koganei, Tokyo, Japan
Keywords:
Geometric Phase, Berry Phase, Fabry-P
´
erot Cavity.
Abstract:
We have proposed a method to scan resonance modes in a Fabry-P
´
erot cavity applying a geometric phase
shifter, named as spectral drill. When the geometric phase shifter consists of two quarter wave plates and a
half wave plate sandwiched by them is put into a cavity, the resonance modes can be scanned by the rotation
of the half wave plate. Since a mechanical rotation stage has been used for scanning the cavity in our prior
works, the scanning rate was limited by the rotational speed of the stage. In this work, by replacing the half
wave plate to a q-plate with removing the mechanical stage and by taking transmission images by a camera,
we succeeded in 720 times faster acquisition of the transmission spectrum of the spectral drill.
1 INTRODUCTION
Regular separation of resonance modes of a Fabry-
P
´
erot (FP) cavity in the spectral region has been used
as a spectral ruler and has been applied to a frequency
marker for the fine spectroscopy and the essential
principle of a frequency comb(Del’Haye et al., 2009).
Since the frequency separation between the resonance
modes depends on the cavity length d, the resonance
frequency can be scanned by changing the cavity
length. The maximum scanning frequency range in
the FP cavity is restricted by physical limitations of
the cavity mirror position or by the tunable range of
physical properties defining the effective light path
length within the cavity. For instance, one of cavity
mirrors is supposed to be mounted on a piezo manip-
ulator for scanning along optical axis. In such case,
the scanning length is limited to only micron order. It
may be enough length for visible light, but short for
the IR range, in which the wavelength becomes longer
than one micron. Other problem for piezo scanner is
on its hysteresis. For the precise measurement, one
has to take care about the reproducibility of the fre-
quency axis because of a piezo hysteresis.
A geometric phase experienced by a light whose
polarization state moves on a Poincar
´
e’s sphere is
a
https://orcid.org/0000-0002-8414-9181
b
https://orcid.org/0000-0002-2611-882X
c
https://orcid.org/0000-0002-8570-8504
understood as the analogy of the Berry phase ex-
perienced by the wave-function of electron propa-
gating in a vector field. The geometric phase of a
light had been investigated by Puncharatnum in old
days(Pancharatnam, 1956). As an application of the
geometric phase of a light, a geometric phase shifter
(GPS) consists of a simple series of phase plates has
been also proposed by some groups for precise con-
trol of the optical phase(Yang et al., 2010) and the
THz-wave phase(Kawada et al., 2016). Recently, the
geometric phase has attracted many researches be-
cause the metasurface technology can control such
geometric phase of a light by designing artificial
structures(Huang et al., 2012).
We have previously reported an optical configu-
ration named “spectral drill,” in which a GPS is put
into a FP cavity. In the spectral drill, the resonance
frequency of the FP cavity can be swept continuously
with seamless manner by rotating a phase plate con-
sisting the GPS(Ohno, 2018). The motion of reso-
nance modes moving on the spectral region with ro-
tating the phase plate looks like apparent motion of
grooves on a drill with the rotation. This behavior is
responsible for the name of spectral drill. Since, in
our previous system, the phase plate was mounted on
an auto-rotational stage, the scanning speed of the res-
onance modes was limited by the rotational speed of
the mechanical movement. In this paper, we propose
a method to break this limitation by replacing a phase
plate with a q-plate and using a camera.
Ohno, S., Miyamoto, K., Hayashi, S. and Sekine, N.
High Speed Measurement in Spectral Drill using Q-plate and Camera.
DOI: 10.5220/0008959400970099
In Proceedings of the 8th International Conference on Photonics, Optics and Laser Technology (PHOTOPTICS 2020), pages 97-99
ISBN: 978-989-758-401-5; ISSN: 2184-4364
Copyright
c
2022 by SCITEPRESS – Science and Technology Publications, Lda. All rights reserved
97