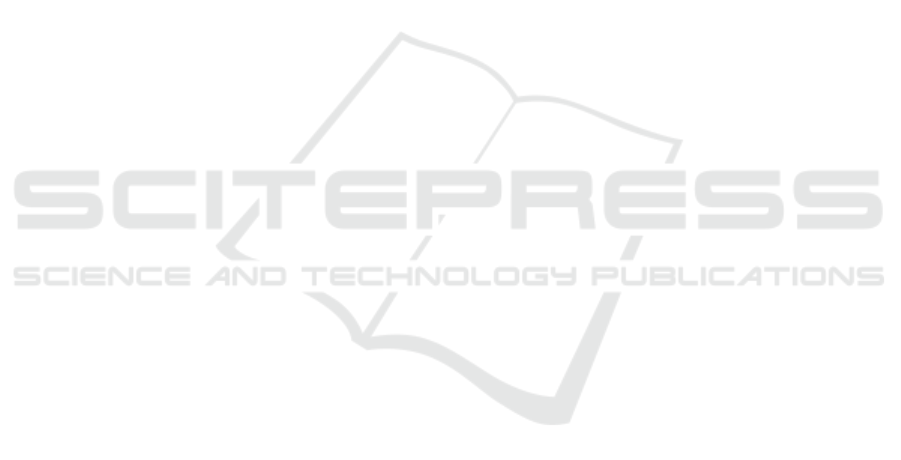
2020 and European Union funds (European Regional
Development Fund)
REFERENCES
Azuma, R. T. (1997). A survey of augmented reality. Pres-
ence: Teleoper. Virtual Environ., 6(4):355–385.
Bachmann, D., Weichert, F., and Rinkenauer, G. (2014).
Evaluation of the leap motion controller as a new
contact-free pointing device. Sensors, 15(1):214–233.
Becerra., D. A. I., Quispe., J. A. H., Aceituno., R. G. A.,
Vargas., G. M. P., Zamora., F. G. F., Mango., J. L. H.,
Figueroa., G. P. A., Vizcarra., A. A. P., and Chana.,
J. W. T. (2017). Evaluation of a gamified 3d vir-
tual reality system to enhance the understanding of
movement in physics. In Proceedings of the 9th Inter-
national Conference on Computer Supported Educa-
tion - Volume 1: CSEDU,, pages 395–401. INSTICC,
SciTePress.
Bekele, M. K., Pierdicca, R., Frontoni, E., Malinverni, E. S.,
and Gain, J. (2018). A survey of augmented, virtual,
and mixed reality for cultural heritage. J. Comput.
Cult. Herit., 11(2).
Bogusevschi., D. and Muntean., G. (2019). Water cycle in
nature – an innovative virtual reality and virtual lab:
Improving learning experience of primary school stu-
dents. In Proceedings of the 11th International Con-
ference on Computer Supported Education - Volume
1: CSEDU,, pages 304–309. INSTICC, SciTePress.
Bonde, M. T., Makransky, G., Wandall, J., Larsen, M. V.,
Morsing, M., Jarmer, H., and Sommer, M. O. (2014).
Gamified laboratory simulations motivate students
and improve learning outcomes compared with tra-
ditional teaching methods. Nature Biotechnology,
32(7):694–697.
Bratitsis, T. and Kandroudi, M. (2014). Motion sensor tech-
nologies in education. EAI Endorsed Transactions on
Serious Games, 1(2).
Brown, S. and Vaughan, C. (2010). Play: How it Shapes
the Brain, Opens the Imagination, and Invigorates the
Soul. J P Tarcher/Penguin Putnam.
Burdea, G. and Coiffet, P. (1993). Virtual Reality Technol-
ogy. NY: John Wiley & Sons, Inc.
d’Aquin, M. (2016). On the Use of Linked Open Data in
Education: Current and Future Practices, pages 3–
15. Springer International Publishing, Cham.
de Vries, L. E. and May, M. (2019). Virtual labora-
tory simulation in the education of laboratory tech-
nicians–motivation and study intensity. Biochemistry
and Molecular Biology Education, 47(3):257–262.
Ebner, M. and Spot, N. (2016). Game-Based Learning
with the Leap Motion Controller, pages 555–565. IGI
Global.
Estapa, A. and Nadolny, L. (2015). The effect of an aug-
mented reality enhanced mathematics lesson on stu-
dent achievement and motivation. Journal of STEM
Education, 16(3):40.
Ferrer-Torregrosa, J., Torralba, J., Jimenez, M. A., Garc
´
ıa,
S., and Barcia, J. M. (2015). Arbook: Development
and assessment of a tool based on augmented reality
for anatomy. Journal of Science Education and Tech-
nology, 24(1):119–124.
Gardelis., K., Lalos., A. S., and Moustakas., K. (2018). De-
velopment of an eco-driving simulation training sys-
tem with natural and haptic interaction in virtual re-
ality environments. In Proceedings of the 13th Inter-
national Joint Conference on Computer Vision, Imag-
ing and Computer Graphics Theory and Applications
- Volume 3 IVAPP: HUCAPP,, pages 94–101. IN-
STICC, SciTePress.
Garz
´
on, V., Carlos, J., Magrini, M. L., and Galembeck, E.
(2017). Using augmented reality to teach and learn
biochemistry. Biochemistry and Molecular Biology
Education, 45(5):417–420.
Heradio, R., de la Torre, L., Galan, D., Cabrerizo, F. J.,
Herrera-Viedma, E., and Dormido, S. (2016). Virtual
and remote labs in education: A bibliometric analysis.
Computers & Education, 98:14 – 38.
Karakasidis, T. (2013). Virtual and remote labs in higher ed-
ucation distance learning of physical and engineering
sciences. In 2013 IEEE Global Engineering Educa-
tion Conference (EDUCON), pages 798–807.
Kiourt, C., Koutsoudis, A., and Pavlidis, G. (2016). Dyna-
mus: A fully dynamic 3d virtual museum framework.
Journal of Cultural Heritage, 22:984 – 991.
Lalos, A. S., Nousias, S., and Moustakas, K. (2018).
Gamecar: Gamifying selfmanagement of eco-driving.
ERCIM News 115 (Oct. 2018): 46-47.
Lalos, S., Kiourt, C., Kalles, D., and Kalogeras, A. (2020).
Personalized interactive edutainment in extended real-
ity (xr) laboratories. ERCIM News, Educational Tech-
nology, 120:29–30.
Liang, J., Su, W., Chen, Y., Wu, S., and Chen, J. (2019).
Smart interactive education system based on wearable
devices. Sensors, 19(15).
Makransky, G., Thisgaard, M. W., and Gadegaard, H.
(2016). Virtual simulations as preparation for lab ex-
ercises: Assessing learning of key laboratory skills
in microbiology and improvement of essential non-
cognitive skills. PLoS One, 11(6).
Mann, S., Furness, T., Yuan, Y., Iorio, J., and Wang, Z.
(2018). All reality: Virtual, augmented, mixed (x),
mediated (x,y), and multimediated reality.
Milgram, P. and Kishino, F. (1994). A taxonomy of mixed
reality visual displays. IEICE TRANSACTIONS on In-
formation and Systems, 12:1321–1329.
Mouromtsev, D. and d’Aquin, M. (2016). Open Data for
Education: Linked, Shared, and Reusable Data for
Teaching and Learning. Springer.
Nicholson, S. (2011). Making gameplay matter : Design-
ing modern educational tabletop games. Knowledge
Quest, 40(1):60–65.
Nicholson, S. (2012). Strategies for meaningful gamifica-
tion: Concepts behind transformative play and partic-
ipatory museums. In Presented at Meaningful Play
2012. Lansing, Michigan.
XRLabs: Extended Reality Interactive Laboratories
607