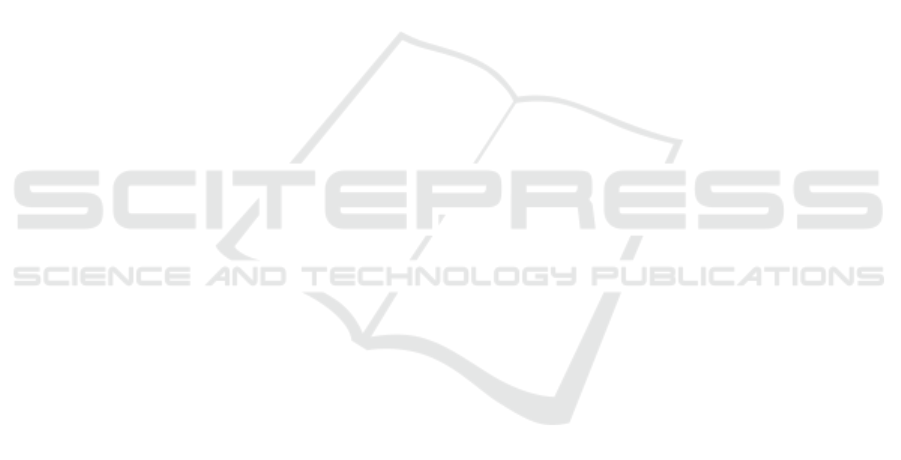
43% during scenario-based hazard analysis (at the
early stage by clustering and grouping) is counted as
an outcome of this paper for supporting a complete
HADF scenario set. Furthermore, using a stochas-
tic program to optimize the safety-relevant parame-
ter’s boundary reduces the number of logical scenar-
ios which reduce the effort of modeling scenarios.
A simple stochastic program is advantageous at the
function level to deal with a large number of scenar-
ios and uphold to sustain a systematic approach that
supports the safety aspect of HADF. This approach
supports in general to build confidence in simulation-
based scenario investigation in HADF development
which can be cost-effective and time-efficient. Al-
though, a further scenario reduction approach is re-
quired from a logical scenario to concrete scenario
determination.
In our future research, we would like to focus on
the complete scenario database for a complete HADF
and propose a reduced set of concrete scenarios based
on simulation results that can support to provide ev-
idence for safety approval (homologation). Approxi-
mation techniques that reduce more scenarios in each
step of clustering and/or grouping at function level
may be improved by other types of stochastic pro-
grams like a multistage program. Interesting is to ob-
serve the accuracy rate of the experiment and simu-
lation result by studying a huge number of scenarios
which has to be investigated.
REFERENCES
Amersbach, C. and Winner, H. (2019). A contribution to
overcome the parameter space explosion during vali-
dation of highly automated driving. Taylor & Francis,
20th edition.
Cana, R., Ferrares, P., Townsend, E., Jost, G., Janitzek, T.,
Pogorelov, E., Popolizio, M., Simcic, G., and Berg,
Y. (2008). Speedfact sheet. German Autobahn: The
Speed Limit Debate.
Damm, W., M
¨
ohlmann, E., and Rakow, A. (2020). Valida-
tion and Verification of Automated Systems. Springer
Nature Switzerland AG., European Union, 1st edition.
Dupa
ˇ
cov
´
a, J. and Kozm
´
ık, V. (2015). SDDP for multistage
stochastic programs: preprocessing via scenario re-
duction. Springer.
Fadhloun, K., Rakha, H., Loulizi, A., and Wang, J.
(2020). A validation study of the fadhloun-rakha car-
following model. In Proceedings of the 6th Interna-
tional Conference on Vehicle Technology and Intel-
ligent Transport Systems - Volume 1: VEHITS,. IN-
STICC, SciTePress.
Galizia, A. D., Bracquemon, A., and Arbaretier, E. (2018).
A scenario-based risk analysis oriented to manage
safety critical situations in autonomous driving. Safety
and Reliability – Safe Societies in a Changing World,
pages 1357–1362.
Gyllenhammar, M., Johansson, R., Warg, F., Chen, D.,
Heyn, H., Sanfridson, M., and Ursing, S. (2020). To-
wards an operational design domain that supports-
the safety argumentation of an automated driving sys-
tem. 10th European Congress on Embedded Real
Time Software and Systems (ERTS2020).
Hassan, S., Puan, O., Mashros, N., and N.Sukor (2014).
Factors affecting overtaking behaviour on single car-
riageway road: Case study at jalan kluang-kulai. Spe-
cial Issue on Highway and Transportation Engineer-
ing Part 1.
Holger, H. and Werner, R. (2003). Scenario Reduction Al-
gorithm in Stochastic Programming. Springer.
Holger, H. and Werner, R. (2009). Scenario tree reduction
for multistage stochastic programs. Springer.
ISO26262 (2018). Road vehicles — Functional safety. ISO,
2nd edition.
ISO/PAS21448 (2019). Road vehicles — Safety of the in-
tended functionality. ISO, 1st edition.
Kalisvaart, S., Slavik, Z., and Camp, O. (2019). Valida-
tion and Verification of Automated Systems. Springer,
Cham., Switzerland, 1st edition.
Khatun, M., Glaß, M., and Jung, R. (2020). Scenario-based
extended hara incorporating functional safety and so-
tif for autonomous driving. 30th European Safety and
Reliability Conference.
Kopestinsky, A. (2021). 25 astonishing self-driving car
statistics for 2021. Policy Advice.
Leither, A., Watzenig, D., and Ibanez, J. (2020). Valida-
tion and Verification of Automated Systems. Springer
Nature Switzerland AG., European Union, 1st edition.
Macher, G., Druml, N., Veledar, O., and Reckenzaun,
J. (2019). Safety and Security Aspects of Fail-
Operational Urban Surround perceptION (FUSION).
IEEE2018, China.
Mazzega, J. (2019). Pegasus method: An overview. PE-
GASDU Symphony.
Menzel, T., Bagschik, G., and Maurer, M. (2018). Scenar-
ios for development, test and validationof automated
vehicles. 2018 IEEE Intelligent Vehicles Symposium
(IV), Changshu.
NHTSA (2017). Automaed Driving Systems-A Vision for
Safety. u.s Depertment of transportation, U.S, 2nd edi-
tion.
SAEJ3016 (2018). Taxonomy and definitions for terms re-
lated to driving automation systems for on-road motor
vehicles. 30th European Safety and Reliability Con-
ference.
VEHITS 2021 - 7th International Conference on Vehicle Technology and Intelligent Transport Systems
308