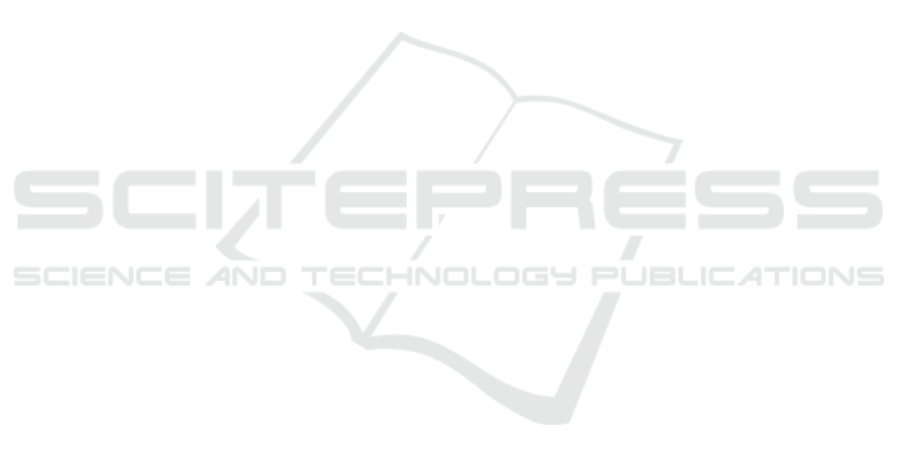
ACKNOWLEDGEMENTS
“This research is co-financed by Greece and the
European Union (European Social Fund- ESF)
through the Operational Programme «Human
Resources Development, Education and Lifelong
Learning 2014-2020» in the context of the project “A
Total Cost of Ownership Model for Automated and
Electric Vehicles (TCO4AEV)” (MIS 5049185).”
REFERENCES
ACEA, 2016. New passenger car registrations by
alternative fuel type in the European Union – Quarter
4 2015, European Automobile Manufacturers
Association. Retrieved from https://www.acea.be/
AIC, 2020. Average age of passenger cars in some
European countries, Automotive Information Centre.
Retrieved from https://www.aut.fi/
Alvarez, 2021. The best-sellinghynrid cars in France
(2020). L’auto journal. Retrieved from https://www.
autojournal.fr/economie/voitureshybrides-plus-vendue
s-france-2020-260958.html#item=1.
AVERE, 2014. Hybride: un marché en recul en 2014,
l'hybride essence tient le coup" (in French). France
Mobilité Électrique.
Bakker, D. 2010. Battery electric vehicles performance,
CO
2
emissions, lifecycle costs and advanced battery
technology development, Master thesis, Sustainable
Development, Energy and Resources Copernicus
Institute, University of Utrecht, Holland.
Benson, A. J., Tefft, B. C., Svancara, A. M., & Horrey, W.
J., 2018. Potential reductions in crashes, injuries, and
deaths from large-scale deployment of advanced driver
assistance systems. AAA Foundation for Traffic Safety.
Bhatti, S.F., M.K., Lim, H.Y. Mak, 2015. Alternative fuel
station location model with demand learning, Annals of
Operations Research, 230, 1, 105–127.
Bösch P.M, Becker, F., Beckerm H., Axhausen, K.W.,
(2018).Cost-based analysis of autonomous mobility
services, Transport Policy, 64, 76-91.
Burch, I., Gilchrist, J., 2018. Survey of global activity to
phase out internal combustion engine vehicles, Center
for Climate Protection.
Carley, S., Krause, R.M., Lane, B.W., Graham, J.D., 2013.
Intent to purchase a plug-in electric vehicle: a survey of
early impressions in large US cities, Transportation
Research Part D: Transport and Environment, 18, 39-45.
Clements, L., Kockelman, K., 2017. Economic effects of
automated vehicles, Transportation Research Record:
Journal of the Transportation Research Board, 2606
(1), 106-114.
Danielis R, Giansoldati M, Rotaris L., 2018. A probabilistic
total cost of ownership model to evaluate the current
and future prospects of electric cars uptake in Italy,
Energy Policy, 119, 268-281.
DeLuchi, M. A., Lipman, T. E., 2001. An analysis of the
retail and life cycle cost of battery-powered electric
vehicles, eScholarship University of California, Davis.
Retrieved from http://escholarship.org/
uc/item/50q9060k.
Duvall, M, 2002. Comparing the benefits and impacts of
hybrid vehicle options for compact sedan and sport
utility vehicles, EPRI.
EAFO project, 2018. The transition to a zero emission
vehicles fleet for cars in the EU by 2050.
EC, 2020. Emergy, Weekly oil bulletin. European
Commission Energy. Retrieved from https://ec.europa.
eu/energy/data-analysis/weekly-oil-bulletin_en?redir=1.
EC, 2018. Emission performance standards for new
passenger cars and for new light commercial vehicles.
European Commission. Retrieved from https://oeil.
secure.europarl.europa.eu/oeil/popups/ficheprocedure.
do?reference=2017/0293(COD)&l=en.
EEA, 2016. Fuel efficiency improvements of new cars in
Europe slowed in 2016, European Environmental
Agency.
Fleet Europe, 2019. New insurance policy launched for
EVs. Retrieved from https://www.fleeteurope.com.
Fleming, K., and Singer, M., 2019. Energy implications of
current travel and the adoption of automated vehicles,
National Renewable Energy Laboratory.
Gaton, 2020. Electric vehicle and hybrid sales hit record
share of 18 per cent in Europe, The Driven. Retrieved
from https://thedriven.io.
Gawron, J. H., Keoleian, G.A., De Kleine, R.D.,
Wallington, T. J., Kim, H.C., 2018. Life cycle
assessment of connected and automated vehicles:
Sensing and computing subsystem and vehicle level
effects, Environmental Science Technology, 52, 5,
3249–3256.
Gnann,T., Plotz,P., 2015. A review of combined models for
market diffusion of alternative fuel vehicles and their
refueling infrastructure, Renewable and Sustainable
Energy Reviews 47, 783–793.
Hagman J, Ritzén S, Stier J, Susilo Y., 2016. Total cost of
ownership and its potential implications for battery
electric vehicle diffusion, Research in Transportation
Business & Management, 18, 11-17.
ICCT, 2016.European vehicle market statistics-Pocketbook
2015/16, International Council on Clean
Transportation. Retrieved from https://theicct.org/
InsideEvs, 2020. How long do electric car batteries last.
Retrieved from https://insideevs.com/features/ 434296/
video-how-long-batteries-last/.
Kockelman, K.M., Lee, J., 2019. Energy implications of
self-driving vehicles, 98th Annual Meeting of the
Transportation Research Board. Transportation
Research Board, Washington D.C.
Lebeau, K., Lebeau, P., Macharis, C., van Mierlo, J., 2013.
How expensive are electric vehicles? A total cost of
ownership analysis, World Electric Vehicle Journal, 6,
996–1007.
Mitropoulos, L.K., Prevedouros, P.D., 2015 Life cycle
emissions and cost model for urban light duty vehicles,
VEHITS 2021 - 7th International Conference on Vehicle Technology and Intelligent Transport Systems
42