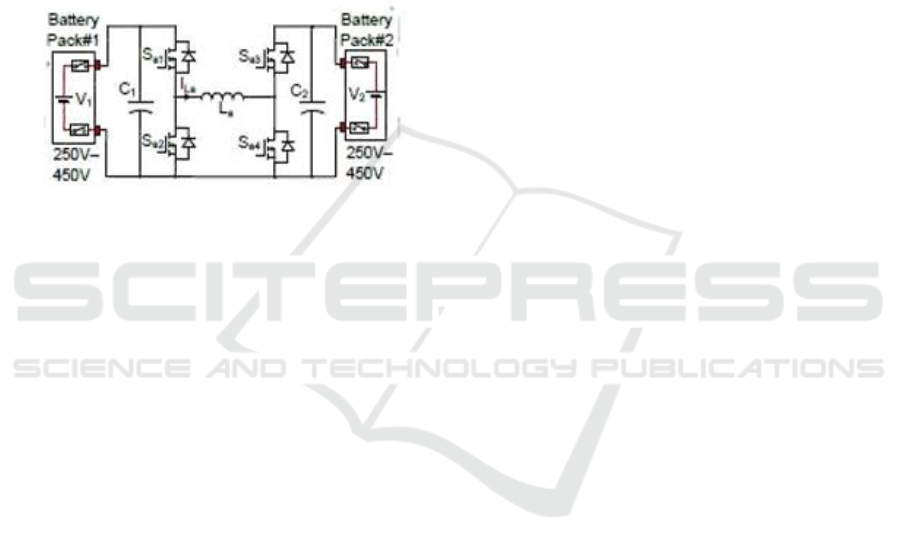
alternative, more convenient, and flexible way of
conducting EV charging.
V2V charging requires an analysis in terms of
how to match suppliers to receivers with efficient
matching algorithms and how to enable energy
exchange with current EV charging technologies
(Mou, et al, 2018). Three different approaches for
V2V energy transfer have been compared in (Sousa,
et al, 2018): vehicle-to-grid and grid-to-vehicle
(V2G+G2V), V2V over direct ac interconnection
(acV2V), and V2V over dc interconnection (dcV2V).
It was concluded that dcV2V is more efficient than
the other options due to reduced number of energy
conversions.
Figure 8: Bidirectional DC-DC converters for V2V charger
(Cuchý, 2018).
The dc-dc converter topologies investigated in this
paper are non-isolated as there is no grid connection
requirement (Cuchý, et al, 2018). One of the
candidate solutions to this operation is known as
bidirectional dc-dc converters is shown in Figure 8.
As a practical industry example, there is a V2V
charging realized by Andromeda Power using ’Orca
Inceptive’ (Andromeda Power, 2020).
7 CONCLUSIONS
In this survey, different EV battery charging systems
are discussed, along with the various battery types
used for different EVs. A theoretical overview on the
various WPT techniques is provided. Multiple WPT
systems are discussed for smart cities. The overview
of each topology is presented. Research is still needed
on the health effects of long-term exposure to weak
electric and magnetic fields, methods to determine
optimal charger power levels and spacing for cost
effectiveness and approaches to analyze impacts of
large-scale WPT system deployment on the electric
grid.
As for future applications, 3 main concepts shall
be considered. First, Wireless vehicle to grid (W-
V2G) which can offer a solution alongside advanced
scheduling for charging and discharging to the
distribution network.
Secondly, in Wheel wireless charging system
(IW-WCS) where receiver coils are placed in a
parallel combination inside the tire. This technology
can rectify air-gap problems. It has been developed
for both stationary and dynamic applications. The
third application is wireless vehicle-to-vehicle
charging technology structure, where the transmitter
coil and the receiver coil are embedded in the front
and rear of the car, respectively. With a limited
number of charging stations, this technology can be
used to increase charging opportunities through
vehicle-to-vehicle (V2V) charging. At present,
charging stations require regular maintenance and
service to ensure the equipment is working properly.
The wireless V2V charging system can help to solve
this issue. The main issue with the wireless V2V
charging technology is the angular offset due to the
change in the location of the vehicle and the reduced
battery size.
REFERENCES
Turksoy, et al., 2018, “Overviewflexible v2v of battery
charger topologies in plig-in electric and hybrid electric
vehicles,” 05 2018.
Bosshard, et al, 2016, “Inductive power transfer for electric
vehicle charging: Technical challenges and tradeoffs,”
IEEE Power Electronics Magazine, pp. 22–30, Sep.
2016.
Freschi, et al, 2018, “Electrical safety of plug-in electric
vehicles: Shielding the public from shock,” IEEE
Industry Applications Magazine, pp. 58–63, May 2018.
Chinthavali, et al, 2016, “All-SiC inductively coupled
charger with integrated plug-in and boost
functionalities for PEV applications,” in 2016 IEEE
Applied Power Electronics Conference and Exposition
(APEC), pp. 1307–1314, IEEE, Mar. 2016.
Cuchý, et al, 2018, “Whole Day Mobility Planning with
Electric Vehicles”. In Proceedings of the 10th
International Conf. on Agents and Artificial
Intelligence – Vol. 2: ICAART, pages 154-164, 2018.
Graber, et al, 2018, “ Centralized Scheduling Approach to
Manage Smart Charging of Electric Vehicles in Smart
Cities”, .In Proceedings of the 7th International
Conference on Smart Cities and Green ICT Systems -
SMARTGREENS, pages 238-245, 2018.
Chentong, et al, 2019, “ Active collision algorithm for
autonomous electric vehicles at intersections”, IET
Intelligent Transport Systems, 2019.
Luigi, et al, 2019, "Power architectures for the integration
of photovoltaic generation systems in DC-microgrids",
Energy Procedia, Volume 159, 2019, Pages 34-41,
A Review on Charging Systems for Electric Vehicles in Smart Cities
577