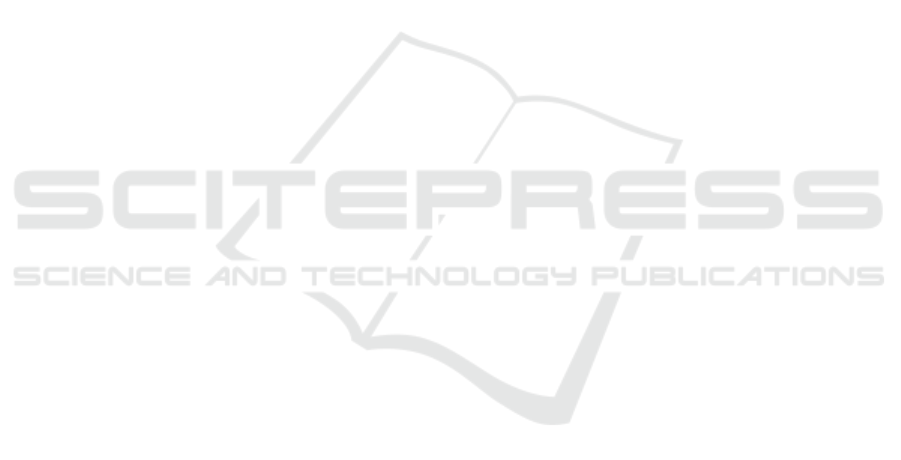
Analysis, ed. B. Stenquist. Stamford, Conn.:
Wadsworth Group, pp. 4–12.
Deng, T., and Nelson, J. D., 2013. Bus rapid transit
implementation in Beijing: An evaluation of
performance and impacts. Research in Transportation
Economics, 39(1): 108–113.
Dion, F., Rakha, H. and Zhang, Y. (2004). Evaluation of
potential transit signal priority benefits along a fixed-
time signalized arterial. Journal of Transportation
Engineering, 130 (3), 294-303.
Garber, N.J., Hoel, L.A., 2008. Traffic and Highway
Engineering, 4th Ed., University of Virginia: United
States of America, Cengage Learning, pp. 99-140.
Gunawan, F.E., Suharjito, Gunawan, A.A.S., 2014.
Simulation Model of Bus Rapid Transit. In: EPJ Web
of Conferences. EDP Sciences 21: 1-7.
Krajzewicz, D., Erdmann, J., Behrisch, M., Bieker, L.,
2012. Recent Development and Applications of SUMO
– Simulation of Urban Mobility. In: International
Journal on Advances in Systems and Measurements, 5
(3 & 4): 128-138.
Lyon Town Planning Agency-LTPA. (2010) Addis Ababa
Bus Rapid Transit Line Feasibility study. Available at:
https://docplayer.net/54352900-Feasibility-study-bus-
rapid-transit-line-addis-ababa.html.
Ngan, V., Sayed, T., Abdelfatah, A., Impacts of Various
Parameters on Transit Signal Priority Effectiveness. In:
Journal of Public Transportation, 7 (3): 71-93.
Papadopoulou, S., Papamichail, I., Roncoli, C., Bekiaris-
Liberis, N., Papageorgiou, M., 2017. Microscopic
simulation-based validation of a per-lane traffic state
esti-mation scheme for highways with connected
vehicles. In: Elsevier Transportation Research Part C,
86 (2018) 441–452.
Papageorgiou, G., Damianou, P., Pitsilides, A., Thrasos, A.,
Ioannou, P., 2006. A Microscopic Traffic Simulation
Model for Transportation Planning in Cyprus. In:
International Conference on Intelligent Systems and
Computing: Theory and Applications, pp. 157-166.
Park, B. and Schneeberger, J.D., 2003. Microscopic
simulation model calibration and validation: case study
of VISSIM simulation model for a coordinated actuated
signal system. In: Transportation Research Record,
1856(1), pp.185-192.
Parr, S.A., Kaisar, E.I., Stevanovic, A., 2014. Application
of Transit Signal Priority for No-Notice Urban
Evacuation. In: ASCE Journal of Natural Hazards
Review 15 (2) :167-170.
Prayogi, L., Satwikasari, A.F., 2019. Bus Rapid Transit-
Oriented Development: An Identification of Bus Rapid
Transit System Passengers’ Modal Shift Potential
Considerations. In: CSID Journal of Infrastructure
Development, (2)1: 127-136.
Raj, G., Sekhar, C.R., Velmurugan, S., 2013. Micro
simulation Based Performance Evaluation of Delhi Bus
Rapid Transit Corridor. 2nd Con. of Transportation
Research Group of India (2nd CTRG). In: Elsevier
Procedia – Social and Behavioural Sciences, 104
(2013) 825-834.
Shaaban, K., Ghanim, M. 2018. Evaluation of Transit
Signal Priority Implementation for Bus Transit along a
Major Arterial Using Microsimulation. The 9th
International Conference on Ambient Systems,
Networks and Technologies (ANT 2018). In: Elsevier
Procedia Computer Science, 130 (2018) 82–89.
Shawn, M.T., William, L.E., Robert, J.B., Douglas, J.H.,
1998. Travel Time Data Collection Handbook: Texas
Transport Institute. The Texas A&M University
System: Collage station, Texas 77843-3135, USA.
Smith, H.R., Hemily, B., Ivanovic, M., 2005 “Transit
Signal Priority (TSP): A Planning and Implementation
Handbook.” ITS America. US Department of
Transportation, Washington DC.
Vedagiri, P., Jain, J., 2012. Simulating Performance
Impacts of Bus Priority Measures. In: ACEE Int. J. on
Transportation and Urban Development, Vol. 2, No. 1,
pp. 15-19.
Wolput, B., Christofa, E., Carbonez, A., Skabardonis, A.,
Tampère, C.M.J., 2015. Optimal Traffic Signal Settings
with Transit Signal Priority. In: Transportation Re-
search Board 94th Annual Meeting Washington D.C.
Ziaei, M., Goharpour, A., 2019. Performance Evaluation of
Tehran Bus Rapid Transport (BRT) Routes by the Two
stage Data Envelopment Analysis (DEA) Model. In:
IJRRAS, 38(1): 87-92.
Transit Performance Evaluation at Signalized Intersections of Bus Rapid Transit Corridors
625