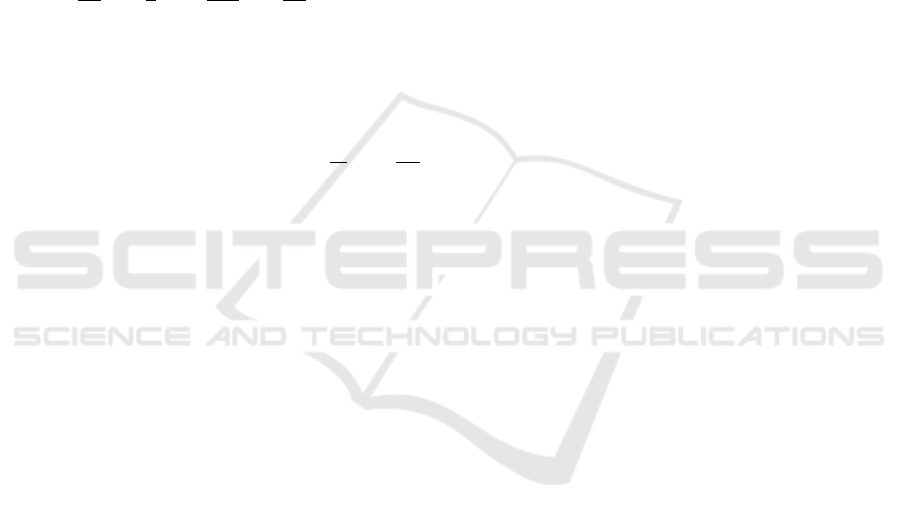
2010). It assumes that the underlying asset price S is
a random variable depending on time t and follow-
ing a geometric Brownian motion (Smoluchowski,
1906). Markets are assumed to be efficient, i.e., the
market movements cannot be predicted (Fama, 1965).
Moreover no arbitrage opportunity, i.e., no risk-free
profit, as well as no transaction cost associated with
the buying or the selling the option contract are as-
sumed (Barles, 1998). Due to the application of Gir-
sanov theorem as well as It
´
o formulas (Weinan et al.,
2019) the stochastic differential equation governing
the stock price volatility is transformed into determin-
istic linear parabolic equation, called Black-Scholes
equation:
∂V
∂t
= −
1
2
(σS)
2
∂
2
V
∂S
2
−rS
∂V
∂S
+ rV, (1)
governing the market price evolution of the European
stock option V =V (t,S) in time interval t ∈[0,T ], T >
0 is given. The short term risk free interest rate r > 0
and the volatility rate σ are assumed to be real con-
stants. The first and second order derivatives of option
price V with respect to S are denoted by
∂V
∂S
, and
∂
2
V
∂S
2
,
respectively. The parabolic equation (1) is completed
by a suitable boundary condition. For the European
call option this boundary condition takes the form:
V (T,S(T )) = max{0,S(T ) −K}, S ∈ [S
0
,S
T
], (2)
where K > 0 is a given strike price at final time t = T
and S
0
= S(0), S
T
= S(T ).
The assumptions of linear Black-Scholes option
pricing model (1)-(2) are simplification of the real
market conditions (Jankova, 2018). Especially the
underlying stock price, its volatility, risk-free inter-
est rate and the stock dividend are unknown, as well
as they may rapidly change in short time interval
with the high variance. This leads to high fluctua-
tions in the calculated option prices with respect to
their market valuation. These discrepancies cause
that researchers and practitioners are looking for new
option pricing models and/or methods. In literature
are described many option pricing models based on
the extension of the classical linear Black-Scholes
model. Among others, there are many non-linear
Black-Scholes models such as Heston models (Hes-
ton, 1993) where the stock volatility or the risk-free
interest rate are assumed to be random rather than
constant functions. The interesting class of option
pricing models are fractional Black-Scholes models
(El Hajaji, 2015), where the stock price is assumed to
follow the geometric L
´
evy process.
The other way to obtain Black-Scholes pricing
model is to use the quantum dynamics methods
(Haven, 2004; Vukovic, 2015; Wr
´
oblewski, 2017).
The similarity between quantum mechanics describ-
ing the micro world of atoms and particles (Einstein,
1924) and stochastic nature of stocks and the associ-
ated options, is based on observation, that quantum
mechanics relies on realization of process generated
by the probability function. In contrary, the stock
price is driven by a stochastic process which is real-
ization of the Brownian motion. These both processes
have just one realization which indicates that there is
a link between probability function and stochastic be-
havior of the stock price. Stock is always traded at
certain prices what is interpreted as the realization of
the stochastic process. The equivalence of the quan-
tum and stochastic descriptions of the stock or op-
tion prices is shown in (Pe
˜
na, 2020; Vukovic, 2015;
Haven, 2004). Especially in (Vukovic, 2015) it is
proved that Black-Scholes equation can be derived
from Schr
¨
odinger equation by using tools of quan-
tum mechanics. In (Haven, 2004) it has been shown
that from the wave equivalent of the Black - Scholes
option price model, a Black – Scholes type option
price with a non-risk free return can be obtained using
Black-Scholes methodology. This quantum approach
has been also used in (Wr
´
oblewski, 2013) where the
linear Black-Scholes equation has been transformed
into linear Schr
¨
odinger equation. We have assumed
that linear Black-Scholes model can be treated as
equivalent to a free quantum particle in constant po-
tential Hilbert space. This observation led to another
approach in (Wr
´
oblewski, 2017), where the solutions
to non-linear Schr
¨
odinger equation have been used for
option pricing. Analytical solutions to the non-linear
Schr
¨
odinger equation with different non-linear poten-
tials, i.e., dark solitons, have been used to calibrate the
model based on the market data. It allows to evaluate
option price (Ivancevic, 2011; Ivancevic, 2010).
This paper is concerned with the development of
new extended non-linear Black-Scholes option pric-
ing model derived from the non-linear Schr
¨
odinger
equation. This model will result from the transforma-
tion of non-linear Schr
¨
odinger equation from Hilbert
into Euclidean space. The obtained Black-Scholes
model will contain additional non-linear terms. Next,
we shall apply method of lines (Sanchez, 2017) to find
numerical solution. Finally we shall calibrate the ob-
tained model using real market data. The paper is
organized as follows. In section 2, we formulate a
non-linear Schr
¨
odinger equation and discuss its fea-
tures. The next section 3 describes the transformation
of this equation into non-linear Black-Scholes equa-
tion. The method of lines and the results of its appli-
cation are presented in section 4. Model calibration
based on the market call options listed on the War-
saw Stock Exchange is provided in section 5. Finally
Non-linear Black-Scholes Option Pricing Model based on Quantum Dynamics
27