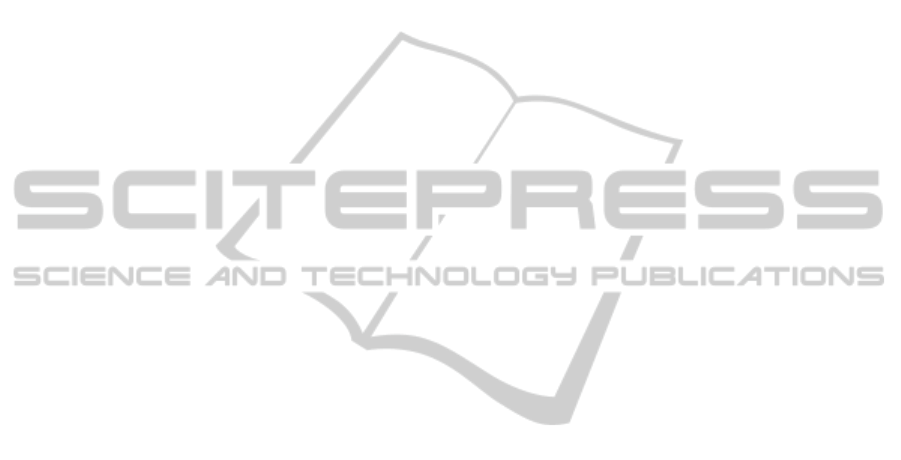
of 0.5 ms duration. The stimulation technique con-
sists of a train of 5 pulses delivered at 1 Hz, which
is equivalent to an interstimulus interval of 100 ms.
Stimulus intensity was gradually increased in incre-
ments of 0.5 mA, starting at 1 mA, up to a maximum
of 3 mA. We testing all the contact with a reference
electrode positioned in the left of grid. During corti-
cal stimulation of the expected motor cortex, move-
ments of distinct portions of the right arm were ob-
served with a stimulation intensity of 2 mA.
4 CONCLUSIONS
The implantable wireless device, here presented, al-
low us to perform chronic EcoG recording and DCS.
research and clinical applications of this novel tech-
nology include brain mapping, seizure foci localiza-
tion and BCI. Wireless technology for transmitting
EcoG signal provides several advantages: risk and
limitation related to the presence of subcutaneous
connecting cables (Behrens et al., 1997) are removed
and the recording time can be substantially prolonged.
This fully-integrated system can also be used as a
closed loop system providing electrical stimulation
“on-demand” to abort promptly detected seizure ac-
tivity in drug-resistant epilepsy patients.
REFERENCES
Behrens, E., Schramm, J., Zentner, J., and K
¨
onig, R. (1997).
Surgical and neurological complications in a series
of 708 epilepsy surgery procedures. Neurosurgery,
41(1):1–10.
Borchers, S., Himmelbach M, Logothetis, N., and Karnath,
H. (2012). Direct electrical stimulation of human cor-
tex: the gold standard for mapping brain functions?
Nature Reviews Neuroscience, 13:63–70.
Cristiani, P., Marchetti, S., Paris, A., Romanelli, P., and
Sebastiano, F. (2012). Implantable device for ac-
quisition and monitoring of brain bioelectric signals
and for intracranial stimulation. WO Patent App.
PCT/IB2012/051,909.
Crone, N. E., Miglioretti, D. L., Gordon, B., and Lesser,
R. P. (1998). Functional mapping of human sensori-
motor cortex with electrocorticographic spectral anal-
ysis. ii. event-related synchronization in the gamma
band. Brain, 121(12):2301–2315.
Johnson, L. A., Wander, J. D., Sarma, D., Su, D. K., Fetz,
E. E., and Ojemann, J. G. (2013). Direct electrical
stimulation of the somatosensory cortex in humans us-
ing electrocorticography electrodes: a qualitative and
quantitative report. Journal of Neural Engineering,
10(3):036021.
Kellis, S., Greger, B., Hanrahan, S., House, P., and Brown,
R. (2011). Platinum microwire for subdural elec-
trocorticography over human neocortex: Millimeter-
scale spatiotemporal dynamics. In Engineering in
Medicine and Biology Society,EMBC, 2011 Annual
International Conference of the IEEE, pages 4761–
4765.
Moro, E., Esselink, R. J., Xie, J., Hommel, M., Benabid,
A. L., and Pollak, P. (2002). The impact on parkinsons
disease of electrical parameter settings in stn stimula-
tion. Neurology, 59(5):706–713.
Rivard, C.-H., Rhalmi, S., and Coillard, C. (2002). In
vivo biocompatibility testing of peek polymer for a
spinal implant system: A study in rabbits. Journal
of Biomedical Materials Research, 62(4):488–498.
Spena, G., Nava, A., Cassini, F., Pepoli, A., Bruno, M.,
DAgata, F., Cauda, F., Sacco, K., Duca, S., Barletta,
L., et al. (2010). Preoperative and intraoperative brain
mapping for the resection of eloquent-area tumors. a
prospective analysis of methodology, correlation, and
usefulness based on clinical outcomes. Acta neu-
rochirurgica, 152(11):1835–1846.
Warren, R. and Durand, D. M. (1998). Effects of applied
currents on spontaneous epileptiform activity induced
by low calcium in the rat hippocampus. Brain Re-
search, 806(2):186 – 195.
NEUROTECHNIX2014-InternationalCongressonNeurotechnology,ElectronicsandInformatics
98