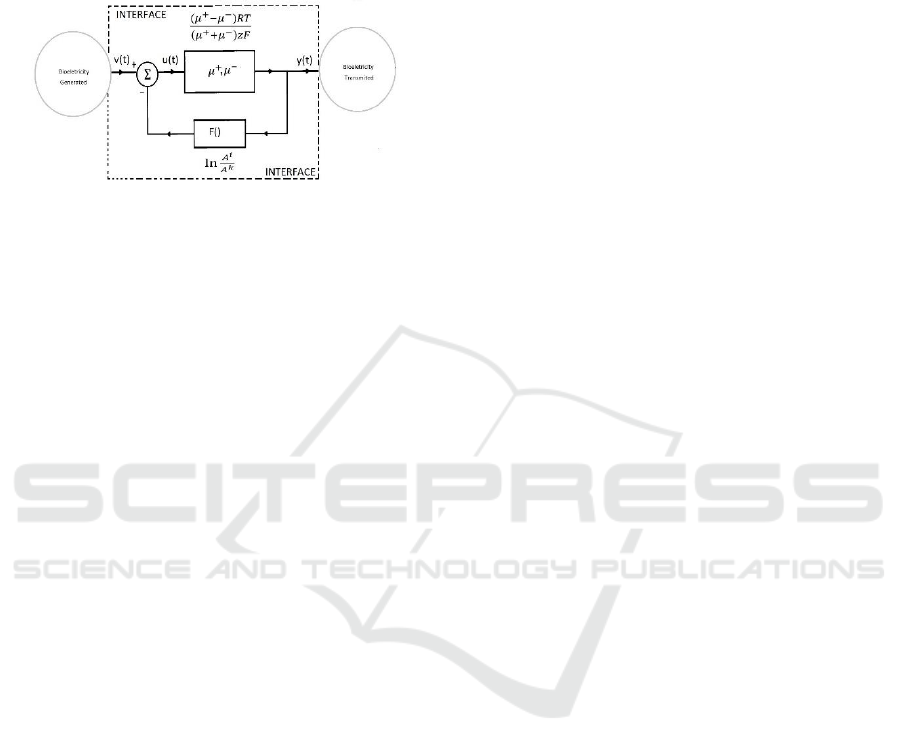
temperature; z represent the system’s valence and F
the system’s Faraday constant.
Based in equation (1) the transduction of signal
from junction is not necessarily linear and therefore
can be modelled as illustrated in Figure 7.
Figure 7: A simple non-linear model proposition for
bioelectricity information transmission in an aqueous
interface.
In the model of Figure (7) the ions activities will
act like a negative feedback function F() and the
coefficients
and
represent the mobility
responsible by the nonlinear transduction in the
interface. The signal transduction between the
interfaces can be described as:
u(t) = v(t) – Fy(t) (2)
F represent the ions activities that act like an
unilateral transference function. In this model, the
distortion of the information caused by the interface
is dependent of how the coefficients
,
and the
ions activities are adjusted.
The results presented in this paper, suggest that de
SINAD is related to several property of the interface,
as described in the literature. When a liquid junction,
i.e, an electrolytic wire, makes the transmission, the
model proposed in Figure 7 can give a direction to the
explanation of why the SINAD of signal transmitted
by electrolytic wire is less than the signal transmitted
by electronic wire. Future works will conducted in
order to test if the model proposed will explain the
data obtained. Overall results in this study allow the
follow scientific question: is electrolytic transmission
the alternative way to send signal in biological
structures? To answer this question more experiments
must be done with different kinds of biological
structures but this start point open a door in the area
of bioelectricity transmission.
7 CONCLUSIONS
A new method for transmission of bioelectricity using
electrolytic wire was presented. The signal distortion
of signal transmitted by electrolytic wire was less
than the signal transmitted by an electronic wire. In
addition, a non-linear model to explain the effect of
aqueous junction was proposed and could explain the
results obtained. Overall results allow to conclude
that in plant tissues the transmission of bioelectricity
is less disturbed than by means of electronic
transmission. This research open a new door in the
area of bioelectricity transmission among biological
structures.
ACKNOWLEDGEMENTS
The author would like to thanks the National Agency
for Research Support CNPq (Proc Num, 311084).
REFERENCES
Agnew, W.F., McCreery, D.B., Yuen, T.G.H., Bullara,
L.A. 1989. Histologic and physiologic evaluation on
electrically stimulated peripheral nerve: considerations
for the selection of parameters. Ann Biomed Eng.
17:39–60.
Cabral, E. F., Pécora, P.C., Arce, A.I.C., Tech, A.R.B.,
Costa, E.J.X., 2011. The oscillatory bioelectrical signal
from plant explained by a simulated electrical model
and tested using Lempel-Ziv Complexity. Computers
and Electronics in Agriculture. 76: 1-5.
Choi, W., Hilleary, R., Swanson, J., Kim, S.H., Gilroy, S.,
2016. Rapid, long-distance electrical and calcium
signalling in plants. Annu. Rev. Plant. Biol. 67:287-
307.
Clement, R.G.E., Bugler, K.E., Oliver, C.W., 2011. Bionic
prosthetic hands: A review of present technology and
future aspirations. The Surgeon, vol.9, 336–340.
Enderle, J.D., Bronzino J., 2011. Introduction to
Biomedical Engineering. 3 rd Edition, ISBN:
9780123749796.
Farina, D., Merletti, R., Enoka, R.M., 2004. The extraction
of neural strategies from the surface EMG. Journal of
Applied Physiology. 96(4):1486-1495.
Freire, F C M., Becchi, M., Pontil, S., Miraldi, E., Strigazzi,
A., 2010. Impedance spectroscopy of conductive
commercial hydrogels for electromyography and
electroencephalography. Physiol. Meas. 31:S157–
S167.
Fromm, J., Hajirezaei, M., Becker, V.K., Lautner, S., 2013.
Electrical signalling along the phoem and its
physiological responses in the maize leaf. Front Plant
Sci. 4:1-7.
Grigoriev, V.A., Kharin, V.N., 2011. An estimate of the
error of measuring sensitivity by the SINAD method.
Measurement Techniques, v 54, n 5, p 544-553.
Horno, J., Castilla, J., Gonzalez-Fernandez., C. F. 1992. A
new approach to nonstationary ionic transport based on
Electrolytic Wire as an Alternative Bio Interface: A Case Study in Plant Tissue
137