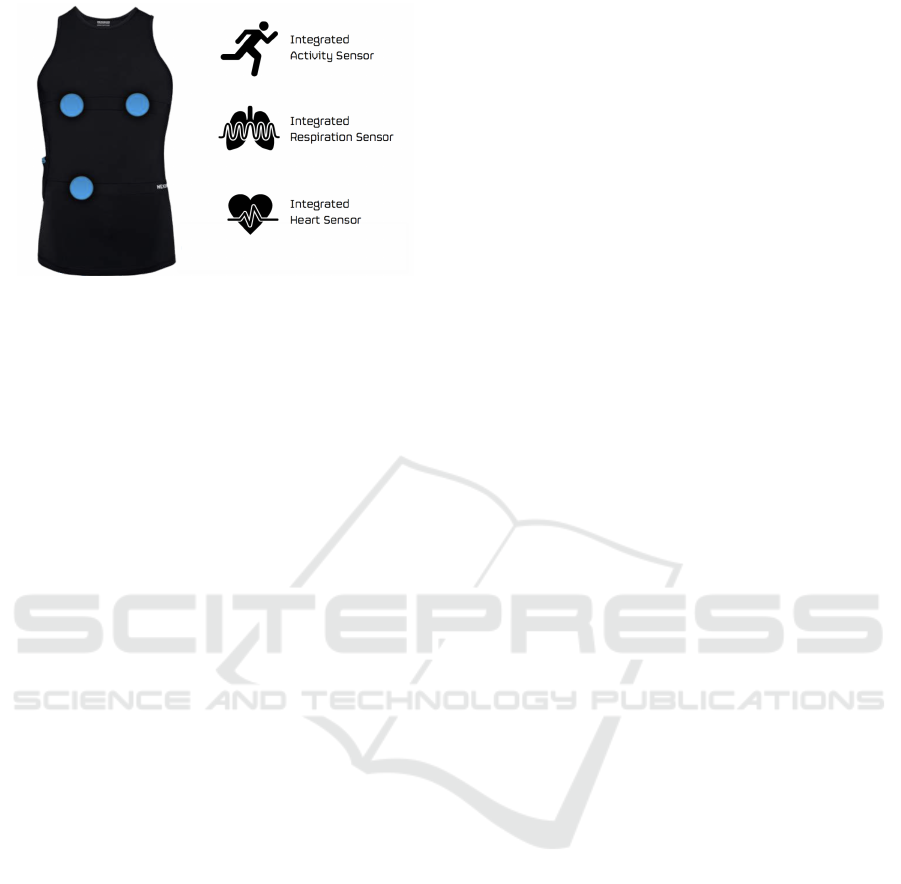
Figure 1: The Hexoskin acquisition system: (a) Activity
sensor, (b) Respiration sensor and (c) Heart sensor.
tidal volume and minute ventilation from the Hex-
oskin closely tracked those measured by the labora-
tory standard method. In another study, to measure
cardiorespiratory fitness, the Hexoskin has been used
in a sample of 10 participants using a hiking situa-
tion during two field activities at different intensities
(Montes et al., 2015). No significant differences for
trail types were noted for maximal heart rate and res-
piratory rate. This is due to many missed data dur-
ing the data collection. In a recent study, to moni-
tor heart rate, twelve male volunteers wore the Hex-
oskin and Polar H7 heart rate sensor and they com-
pleted variable physical activities, using a stationary
training bicycle under two different climate condi-
tions (Al Sayed et al., 2017). The study revealed a
high correlation and an absence of significant differ-
ences between the two systems in monitoring the sub-
jects’ heart rates. In another study, to investigate the
validity and reliability of the Hexoskin vest for mea-
suring respiration and heart rate, ten male elite cy-
clists conducted a maximal aerobic cycle ergometer
test using a ramped protocol (Elliot et al., 2017). Au-
thors conclude that the Hexoskin vest is sufficiently
valid and reliable for measurements of breathing fre-
quency and heart rate in elite athletes but the calcu-
lated minute ventilation value produces during such
exercise should be used with caution due to the lower
validity and reliability of this variable. Finally, the
maximum oxygen uptake (VO
2max
) has been evalu-
ated by (Bassett Jr and Howley, 2000; Hawkins et al.,
2007). This parameter, which can be defined as the
maximum amount of oxygen that the body consumes
during an intense effort (Howley et al., 1995), is con-
sidered as the best indicator of cardiorespiratory func-
tion. Moreover, an important parameter in determin-
ing the quality of a medical instrument is the agree-
ment with a gold standard. These previous studies
have been performed on a small sample size which
can seriously limit the interpretation of the results and
the validation process. In addition, in some cases
(Al Sayed et al., 2017; Elliot et al., 2017), the data col-
lection has been performed on stationary bikes which
does not take into account the usual movements of the
upper body.
In this study, we compared cardiorespiratory pa-
rameters from the Hexoskin against standard labo-
ratory devices during a progressive maximal exer-
cise test. Therefore, the purpose was to validate car-
diorespiratory parameters measured simultaneously
by Hexoskin and a laboratory standard devices dur-
ing a progressive maximal exercise test according
to BSU/Bruce ramp (Kaminsky and Whaley, 1998)
protocol. More precisely, we will compare heart
rate (HR) from the Hexoskin and heart rate from a
reference laboratory electrocardiogram (ECG). The
breathing rate (BR) and the estimated ventilation (Ve)
will be compared between Hexoskin and a reference
laboratory metabolic cart.
2 METHODOLOGY
2.1 Participants
Thirty healthy volunteers (18 males and 12 females)
participated in the study. For 2 participants, the mea-
surements were removed from the database due to
technical issues that occurred when recording of the
sensors signals that were not in the correct format.
The problem was reported to Hexoskin for investi-
gation. This gave a total of twenty-eight volunteers
(17 males and 11 females, age 27.07± 7.25 years old,
weight 73.73 ± 13.41 kg, heigh 173.12 ± 8.15 cm,
Body Mass Index BMI 24.5 ± 3.44).
The study was approved by institutional ethics
committees, with all subjects providing written in-
formed consent before their participation. All partic-
ipants completed a sociodemographic questionnaire
and the Q-AAP + questionnaire to confirm that they
were able to perform a vigorous exercise.
2.2 Data Collection
Data collection was performed at the University of
Sherbrooke exercise physiology laboratory during a
progressive maximal exercise test. Each participant
wore the smart textile Hexoskin while they were sub-
mitted to a progressive maximal exercise test using
the Cosmed Quark metabolic cart and 12 lead elec-
trocardiogram which are considered gold standard in
an exercise physiology lab.
The test was performed according to the
BSU/Bruce ramp protocol (Kaminsky and Whaley,
1998), a testing protocol commonly used in clinical
Physiological Data Validation of the Hexoskin Smart Textile
151