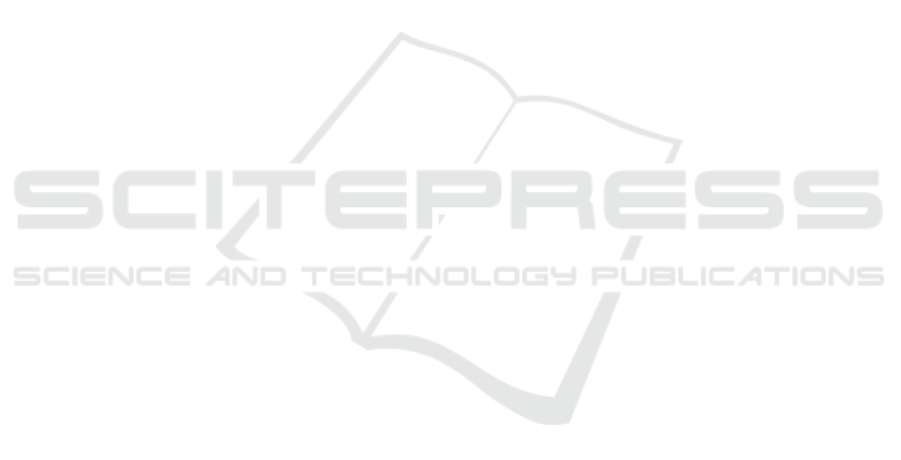
Simultaneous Measurement of a Blood Flow and a Contact Pressure
Ryo Inoue
1
, Hirofumi Nogami
2
, Eiji Higurashi
3
and Renshi Sawada
2
1
Graduate School of Systems Life Sciences, Kyushu University, Japan
2
Department of Mechanical Engineering, Kyushu University, Japan
3
Department of Precision Engineering, University of Tokyo, Japan
Keywords: Optical Sensor, Blood Flow, Contact Pressure, Laser Doppler, MEMS.
Abstract: Although a number of laser Doppler blood flow sensors have been developed over the past few decades, they
remain uncommon in practice. This is because the contact pressure between the skin and the sensor is not
measured simultaneously with blood flow, despite the fact that blood flow is greatly affected by contact
pressure. Thus, reliable and highly reproducible measurement of blood flow could not yet be realized. In
addition, changes in beam conditions or body temperature also have an effect on blood flow measurement.
Therefore, we fabricated a micro electro mechanical system (MEMS) blood flow sensor which can measure
contact pressure, beam power, and body temperature, for reliable and highly reproducible measurement.
1 INTRODUCTION
Continuous health monitoring systems using
biomedical sensors have been widely studied recently
to prevent certain diseases. Laser Doppler blood flow
meter has been studied to enhance health monitoring
systems because the monitoring of peripheral blood
flow just beneath the skin is intimately correlated
with health conditions and the nervous systems
(Wolfman Doehner et al., 2002. Julian M. Stewart et
al., 2004. M. Yasushi et al., 2003).
The laser Doppler flow meter is a non-invasive
method for measuring blood flow in the micro
circulation of biological tissue, and was established by
Stern et al. in 1977 (Stern, M. D. et al., 1977). The
device has been widely used in clinical medicine,
microcirculation, dermatology, and autonomic
function research. There are commercialized blood
flow meters such as the ADMEDEC Laser Doppler
blood flow meter (ALF21; ADVANCE CO., LTD),
stationary and optical fiber type blood flow meters
(OMEGAFLO; OMEGAWAVE, INC), and fiber-less
blood flow meter (RBF-101; Pioneer Corporation).
Moreover, the development of blood flow meters using
micro electric mechanical systems (MEMS)
techniques are currently the focus of active study to
achieve the goals of low power consumption, downsize
the dimensions of the sensor, and maintain low cost. In
an earlier study, the present authors fabricated an
integrated sensor for use in laser Doppler blood flow
measurement systems without optical fibres by
mounting laser diode and photo diode on silicon
substrate (E. Higurashi et al., 2003). Since then,
smaller blood flow sensors have been fabricated using
system line or wafer level packaging techniques (W.
Iwasaki et al., 2010. Y. Kimura et al., 2010).
Although a number of blood flow sensors have
been developed over the past few decades, they are
used in only medical field. This is because contact
pressure has not been considered while measuring
blood flow, despite the fact that blood flow is greatly
affected by it.
Figure 1 shows blood flow when contact pressure
is increased every 20 mmHg. When contact pressure
is lower (0-40 mmHg), the blood flow is larger and
the blood amplitude is smaller. Blood flow decreases
a little, but the amplitude increases when contact
pressure is between 60mmHg and 80mmHg. Finally,
blood flow is about one tenth of what is measured in
the 0mmHg case, and the amplitude decreases again
when it exceeds 100 mmHg. This is because the skin
tissue and vessels are elastic and their deformation via
contact pressure can change the blood stream. Contact
pressure increases linearly, but blood flow and
amplitude change in a non-linear fashion. Furthermore,
the influence of contact pressure on blood flow
measurement is dependent on individual differences
such as the hardness of the skin tissue and blood
pressure. Therefore, simultaneous measurement of
contact pressure is important for optimizing blood flow
measurement.
48
Inoue, R., Nogami, H., Higurashi, E. and Sawada, R.
Simultaneous Measurement of a Blood Flow and a Contact Pressure .
DOI: 10.5220/0006596800480053
In Proceedings of the 11th International Joint Conference on Biomedical Engineering Systems and Technologies (BIOSTEC 2018) - Volume 4: BIOSIGNALS, pages 48-53
ISBN: 978-989-758-279-0
Copyright © 2018 by SCITEPRESS – Science and Technology Publications, Lda. All rights reserved