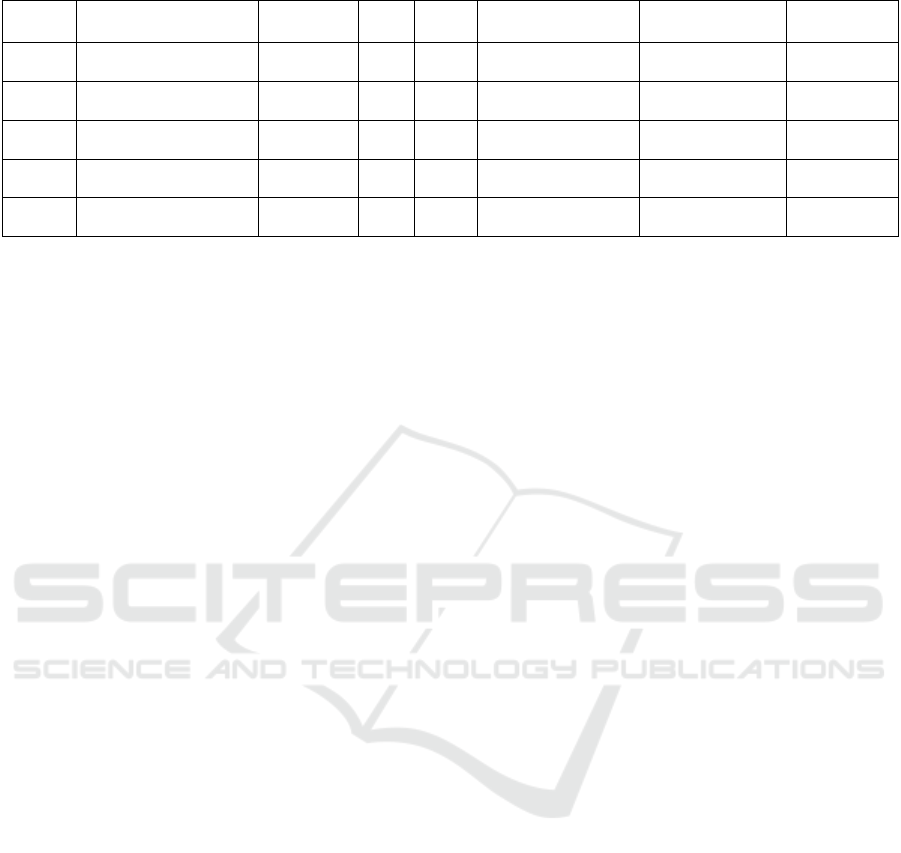
Table 3: Comparison between 2ABD and AGD values in different clinical situations.
could be easily computed and employed as dosimetric
index for each mammographic procedure (i.e. for
each patient) and recorded in the radiological report.
In addition, the computation could be conveniently
automated and this could be advantageous in order to
comply with the European Directive
59/2013/EURATOM. Notice that k
a,i
can be
computed directly from Eq. 2 once kVp, mAs, FID
and t are known and therefore, to assess 2ABD, a
direct measurement of k
a,i
can be
avoided.Furthermore, Table 3 does not show evident
discrepancy between AGD and 2ABD values,
although a different set of radiation exposure and
patient-specific parameters was involved in each
mammographic procedure. Thus, 2ABD could be
employed as surrogate of AGD.
However, our model has some limitations. In fact,
this model could be improved taking into account the
X-ray tube yield variations for different anode-filter
combinations among different mammographic
devices, which can affect the k
a,i
evaluation.
Moreover, breast composition should be considered
and a correction factor might be applied for the μ
en
assessment. In addition, different phantoms with
different shapes could be used so as to better simulate
the breast. Besides, in order to comprehensively
validate this model, the method should be tested in
different clinical conditions on different
mammographic devices. Moreover, breast
composition should be considered and a correction
factor might be applied for the μ
en
assessment. Breast
density is being studied in many epidemiological
works also related to screening programs (Freer 2015,
Berg 2016). We are also realizing image processing
software able to automatically analyse clinical images
to evaluate the breast composition, based on both a
classical approach (comprising a pre-processing step,
a pattern recognition step, a classification step and a
segmentation step) and novel approaches based on
machine learning methods.
As a further development, this method could be
also applied to the tomosynthesis, an advanced
imaging technique that allows the reconstruction of a
three-dimensional view of the breast, overcoming the
projective (two-dimensional) imaging approach
limitations.
5 CONCLUSIONS
In conclusion, in this work we proposed the 2ABD as
a reproducible and easily computable dose index to
assess the radiation dose absorbed by the patient
during a mammography procedure. According to our
preliminary results, the 2ABD could be employed as
dosimetric index to be inserted into the radiological
report as required by the European Directive
59/2013/EURATOM. The development of this new
dose index is a part of a whole project finalized to
optimize the dose in mammographic procedures, give
a correct information about the risk related to ionizing
radiation and maintain high adherence to screening
programs.
REFERENCES
DeSantis, C., Ma, J., Bryan, L., Jemal, A., 2014. Breast can-
cer statistics, 2013. CA Cancer J Clin. Jan-Feb;
64(1):52-62.
Ferlay, J., Héry, C., Autier, P., Sankaranarayanan, R.,2010.
Global Burden of Breast Cancer. Breast Cancer Epide-
miology; 1:1-19.
Dance, D., R., Christodes, S., Maindment, A., D., A.,
McLean, I., D., Ng, K., H., 2014. Diagnostic Radiology
Physics. A Handbook for Teachers and Students. IAEA
International Atomic Energy Agency.
Myers, E., R., Moorman, P., Gierisch, J., M., Havrilesky.,
L., J., Grimm, L., J., Ghate, S., Davidson, B.,
Mongtomery, R., C., Crowley, M., J., McCrory, D., C.,
Kendrick, A., Sanders, G., D., 2015.Benefits and Harms
of Breast Cancer Screening: A Systematic Review.
JAMA Oct 20;314(15):1615-34.
European guidelines for quality assurance in breast cancer
screening and diagnosis 4rd edition, EUREF 2006.
BIODEVICES 2018 - 11th International Conference on Biomedical Electronics and Devices
216