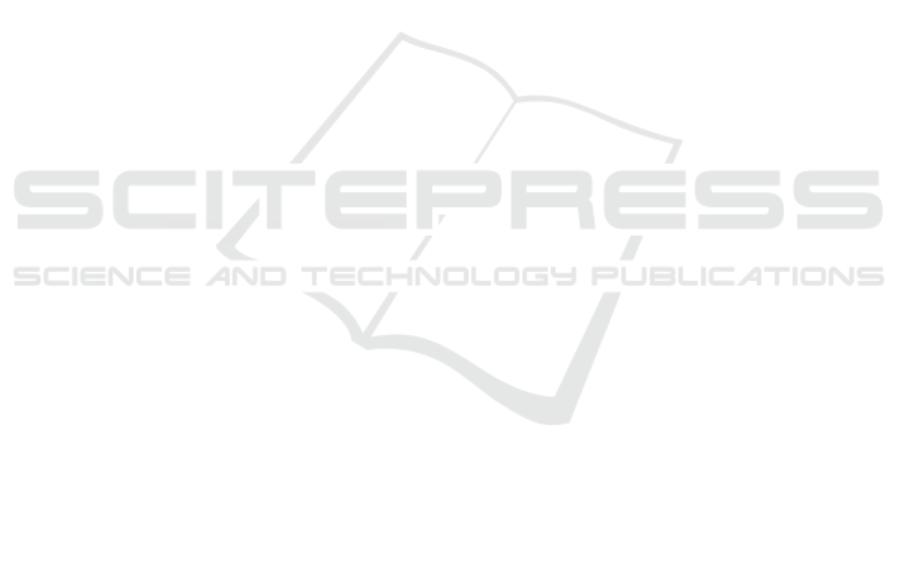
3.2 “Simultaneous Quench” and
“Un-Simultaneous Quench”
Considering the competitive adsorption between two
kinds of fluorophores, it was admired that if the
addition order of two fluorophores alters the
quenching equilibrium, because sometimes the
multi-detection application cannot satisfy the
“simultaneous quench” condition. Here three
experiment schemes were designed, e.g., 1) 2 µL Ur
and 2 µL RB were mixed and diluted firstly, then 10
µL of SWNT-T30 was added (the final mixture
volume was 2 mL), set for 30 min and the
fluorescent intensity of Ur and RB was measured
respectively; 2) 2 µL Ur and 10 µL SWNT-T30 was
mixed and diluted, set for 30 min; then 2 µL RB was
added, set for 30 min (the final mixture volume was
2 mL) and the fluorescent intensity was measured; 3)
2 µL RB and 10 µL SWNT-T30 was mixed and
diluted, set for 30 min; then 2 µL Ur was added into
mixture, set for 30 min (the final mixture volume
was 2 mL) and the fluorescent intensity was
measured. Each experiment above was carried out in
10 times for determination. The final fluorescent
intensity and quenching performance of Ur and RB
in three systems were listed in Table 2.
The final Ur intensity after quenched by SWNT-
T30 from ① “simultaneous added” model was
calculated as 1541 ± 57, less than those from “un-
simultaneous added” model (② and ③,1441 ± 41
and 1445 ± 20). In order to confirm if the 3
distributions could be regarded from the same one,
T-test analysis was carried out for paired samples of
assay ① and ②, ① and ③, ② and ③, respectively.
P values (the freedom degree) were extracted as
0.8279, 0.7014 and 0.6388, all above 0.05, the
threshold of statistical significance, demonstrated Ur
performed similar quenching property in the three
quenching situations. Different results were gained
for RB, an average intensity of 4656 ± 165 in ①
“simultaneous added” model was more than those of
4105 ± 137 in ② “Ur added first” model and 4019 ±
105 in ③ “RB added first” model. The P value of
0.8818 gained via comparing the two “un-
simultaneous added” models ②/③, larger than 0.05,
suggested that there was not difference for optical
properties of RB in multi-fluorophore quenching
systems in which whether RB was quenched first or
not. However, extremely low P values, 1.71E-06 and
9.39E-08, obtained by comparing ① “simultaneous
added” model with “un-simultaneous added” model
(② or ③), indicated the independent photo-
performance of RB in two models; more RB
molecules attached to the SWNT surface and lower
photo-luminescence was observed in the “un-
simultaneous added” model.
Thus, Ur showed similar quenching performance
in both “simultaneous added” model and “un-
simultaneous added” model. For RB, it was
seemingly indicated that in “un-simultaneous added”
model, SWNT-T30 could not quench RB as much as
“simultaneous added” model; while in two “un-
simultaneous added” model, the RB added sequence
had no effects on the quenching performance of RB.
We made a hypothesis that the adsorption of
fluorophore onto SWNT surface was a dynamic
balance process, equal number of fluorophore
molecules attach onto and detach from SWNT
surface at the same time. In the “un-simultaneous
added” model, for example, before RB added, there
had been an equilibrium state of adsorption and
desorption of Ur onto SWNT surface; when RB
added into the quenching system, several sites of Ur
was replaced by RB during the Ur molecules
departed from SWNT surface. Perhaps RB exhibited
stronger scramble ability over Ur for SWNT surface
occupation, which could also be verified by the
quenching efficiency result of single- and multi-
fluorophore quenching system (see section 3.1 and
Table 1), RB showed slighter higher quenching
efficiency in multi-system than single-system while
Ur appeared a contrary tendency.
Moreover, RB itself seemed attached onto
SWNT surface easier in the “un-simultaneous
added” model than “simultaneous added” model. It
was try to be explained that in “simultaneous added”
model, Ur and RB molecules collided more
intensely, so that the attaching probability on SWNT
surface decreased steadily. Also in section 3.1 and
Table 1, RB showed slight higher coverage onto
SWNT surface in competitive system (or multi-
system) than un-competitive system (or single-
system), we thought perhaps it was a little difficult
for RB molecules detach from SWNT surface
because they were blocked by the Ur molecules
surrounded.
As far as the unchanged Ur appearance in all the
three quenching situations, it was proposed that
perhaps there was tiny differences, but the violation
could be ignored, as Ur exhibits a strong quench-
restrain ability to SWNT surface, based on our
previous work.
3.3 Raman Feature
Raman spectra of SWNT-T30 (black), SWNT-
T30/Ur (blue) and SWNT-T30/RB (red) were
measured for evaluating the effects of fluorophores
BIODEVICES 2018 - 11th International Conference on Biomedical Electronics and Devices
234