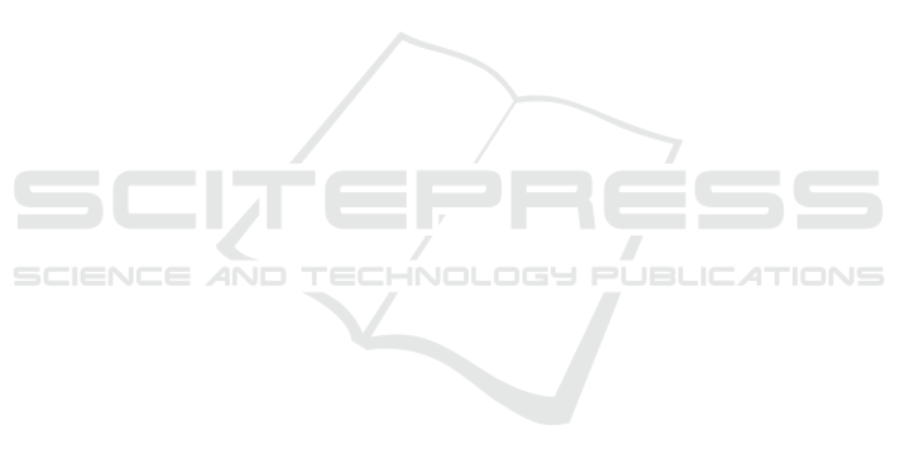
which generate overlapping electrochemical signals
that are hard to distinguish.
To solve this issue and provide an accurate and
meaningful profile of the redox molecules in
biofluids, previous studies suggested modifying
electrodes with films that provide various
reactivities between the electrode and the redox
molecules based on the films’ properties, i.e., their
electrocatalytic activity [e.g., noble metals as
electrode material or conductive polymers to coat
the electrodes (Wei, Yang et al., 2018)] or their
biofunctionality [e.g., enzymes immobilized on the
electrode (Cetó, Voelcker et al., 2016)]. Indeed, such
approaches have enabled, for the first time, profiling
a predetermined set of redox molecules—e.g., for
identifying the redox state of specific molecules
involved in a specific type of cancer [namely,
prostate cancer (Pascual, Campos et al., 2016)].
However, despite this marked advancement in the
ability to differentiate between groups of specific,
disease-oriented ‘redox landscapes’, this approach is
currently lacking the ability to extract redox
information from all redox molecules present in a
biofluid at a given time, hence limiting the diagnosis
to a specific biomedical condition.
3 CONCLUSIONS
This paper highlights the potential contribution of
intelligent multi-sensor arrays for the important need
to profile the ‘redox landscape’ in the body. By
overcoming the fundamental challenge of selectivity
in biofluids, these intelligent sensors will enable the
rapid and continuous quantitative analyses of redox
information in biofluids. Such an achievement is
highly beneficial for a wide range of biomedical
applications, from in vivo diagnostics to in situ
monitoring of cell metabolism, and will offer the
next generation of diagnostic biodevices that can be
used to study and monitor disease initiation and
development. Ultimately, integrating these
intelligent sensors in electrochemical lab-on-a-chip
biodevices will facilitate the development of highly
sensitive monitoring tools for the continuous in vivo
monitoring of various biomedical conditions.
REFERENCES
Bala, A. and Gorski, L., 2016. Application of nucleic acid
analogues as receptor layers for biosensors. Analytical
Methods 8(2): 236-244.
Bandodkar, A. J., Jia, W. Z. and Wang, J., 2015. Tattoo-
based wearable electrochemical devices: A review.
Electroanalysis 27(3): 562-572.
Bandodkar, A. J. and Wang, J., 2014. Non-invasive
wearable electrochemical sensors: A review. Trends in
Biotechnology 32(7): 363-371.
Bard, A. J. and Faulkner, L. R., 2001. Electrochemical
Methods: Fundamentals and Applications, Wiley.
Bougrini, M., Florea, A., Cristea, C., et al., 2016.
Development of a novel sensitive molecularly
imprinted polymer sensor based on
electropolymerization of a microporous-metal-organic
framework for tetracycline detection in honey. Food
Control 59: 424-429.
Bunyakul, N. and Baeumner, A. J., 2015. Combining
electrochemical sensors with miniaturized sample
preparation for rapid detection in clinical samples.
Sensors 15(1): 547-564.
Cetó, X., Voelcker, N. H. and Prieto-Simón, B., 2016.
Bioelectronic tongues: New trends and applications in
water and food analysis. Biosensors and
Bioelectronics 79: 608-626.
Chan, E. C. Y., Pasikanti, K. K., Hong, Y. J., et al., 2015.
Metabonomic Profiling of Bladder Cancer. Journal of
Proteome Research 14(2): 587-602.
Chandrasekaran, A., Idelchik, M. D. S. and Melendez, J.
A., 2017. Redox control of senescence and age-related
disease. Redox Biology 11: 91-102.
Cipri, A., Schulz, C., Ludwig, R., et al., 2016. A novel
bio-electronic tongue using different cellobiose
dehydrogenases to resolve mixtures of various sugars
and interfering analytes. Biosensors and
Bioelectronics 79: 515-521.
Corrie, S. R., Coffey, J. W., Islam, J., et al., 2015. Blood,
sweat, and tears: Developing clinically relevant
protein biosensors for integrated body fluid analysis.
Analyst 140(13): 4350-4364.
Egea, J., Fabregat, I., Frapart, Y. M., et al., 2017.
European contribution to the study of ROS: A
summary of the findings and prospects for the future
from the COST action BM1203 (EU-ROS). Redox
Biology 13: 94-162.
Fekete, S., Guillarme, D., Sandra, P., et al., 2016.
Chromatographic, electrophoretic, and mass
spectrometric methods for the analytical
characterization of protein biopharmaceuticals.
Analytical Chemistry 88(1): 480-507.
Górski, Ł., Kubiak, W. W. and Jakubowska, M., 2016.
Independent components analysis of the overlapping
voltammetric signals. Electroanalysis 28(7): 1470-
1477.
Griffiths, H. R., Gao, D. and Pararasa, C., 2017. Redox
regulation in metabolic programming and
inflammation. Redox Biology 12: 50-57.
Heikenfeld, J., 2016. Non-invasive analyte access and
sensing through eccrine sweat: Challenges and outlook
circa 2016. Electroanalysis 28(6): 1242-1249.
Hong, T. T., Yang, X., Xu, Y. J., et al., 2016. Recent
advances in the preparation and application of
Intelligent Multi-sensor Arrays for Next Generation Diagnostic Biodevices
239