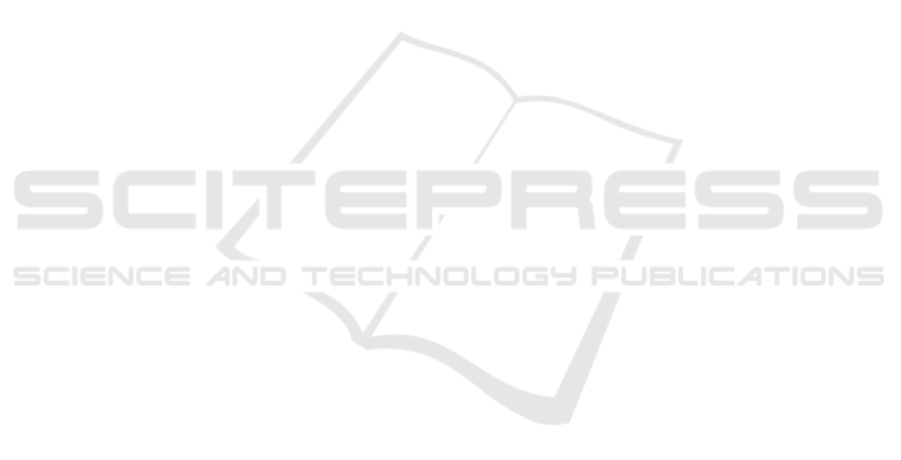
Hydro Chemical Assessment of Edipsos Geothermal Area,
Greece
G
Diamantopoulos
1,*
, D
Poutoukis
2
, B
Raco
3
, A Arvanitis
4
and E
Dotsika
1
1
Stable Isotopes Unit, N.C.S.R. “Demokritos”, Institute of Nanoscience and
Nanotechnology, 15310, Ag. Paraskevi Attikis, Greece
2
General Secretariat for Research and Technology, Mesogion 14-18, 11510, Athens,
Greece
3
Institute of Geosciences and Earth Resources, Via G. Moruzzi 1, 56124 Pisa, Italy
4
Institute of Geology and Mineral Exploration, (I.G.M.E.), S. Loui 1, 3rd entrance of
Olympic Village, 13677, Athens, Greece
Corresponding author and e-mail: G
Diamantopoulos,
g.diamantopoulos@inn.demokritos.gr
Abstract. A geochemical survey on the thermal flu ids of Ed ipsos area was undertaken. In
order to investigate the mineralization process, a geochemical and isotopic analysis (major
ions,
18
O,
2
H) was conducted for thermal waters of springs and boreholes. The Edipsos area is
found in the north part of Euboea, south-east of Athens, and is characterized by high salinity
waters. The evaluation of the geochemical data of the thermal waters of Ed ipsos suggests that
they are fed by thermal water mixed with local groundwater and seawater. The most adequate
geothermometers were applied on selected samples for the determination of the deep aquifer
temperature.
1. Introduction
The thermal springs of Edipsos located in North Euboea are well known since ancient times as
“Pausanias”, and were reported by Aristotle and others for their healing attributes. Despite that these
springs were known from antiquity, the origin of the thermal water remains poorly documented.
Dotsika [1] compared different geothermometers to assess the temperature of reservoirs concluded
that Edipsos thermal field are high-enthalpy hydrothermal system.
2. Geology
The geology of northern Euboea (Figure 1) includes at the lower series a Permian–Triassic
volcanoclastic complex. Its basement consists of metamorphic rocks of pre-middle to middle
Carboniferous age, which are overlain by shallow marine clastic and carbonate rocks of middle
Triassic age [2, 3]. The sedimentary rocks are intercalated with volcanic rocks that are overlain by
Jurassic limestones. An ophiolitic corps, Late Jurassic–Early Cretaceous, is found above the
limestones [2]. The volcanic rocks are best developed at the southeast part of Edipsos. The lithology
is bedded tuff, fine grained agglomerate and rare ignimbrite. Fluvio-lacustrine deposits (Lower
Miocene to Upper Pliocene) were formed during the earlier neotectonic phases of the region [4, 5].
Diamantopoulos, G., Poutoukis, D., Raco, B., Arvanitis, A. and Dotsika, E.
Hydro Chemical Assessment of Edipsos Geothermal Area, Greece.
In Proceedings of the International Workshop on Environmental Management, Science and Engineering (IWEMSE 2018), pages 253-259
ISBN: 978-989-758-344-5
Copyright © 2018 by SCITEPRESS – Science and Technology Publications, Lda. All rights reserved
253