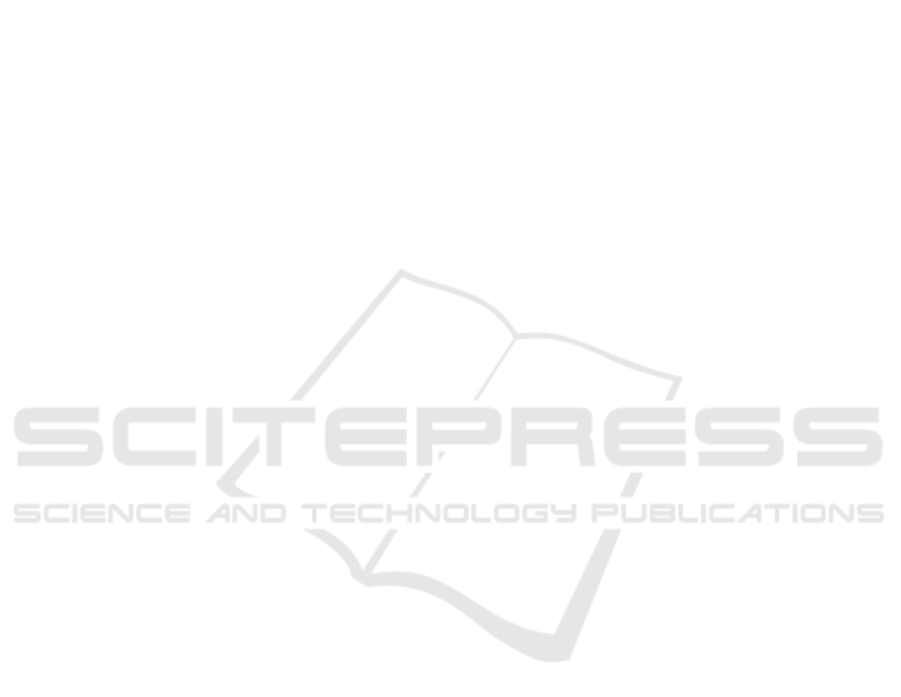
Service Life Design for Infrastructure under Indonesian
Environmental Exposure
Mochamad Hilmy
1
, Herry Prabowo
1
1
Laboratory for Construction, Innovative Structures, and Building Physics,
Department of Architecture, Politeknik Negeri Pontianak, Jl. Ahmad Yani, Pontianak 78124, Indonesia
Keywords: Service Life Design, Infrastructure, Indonesian Tropical Climate, Concrete Durability, Chloride Ingress
Abstract: Reinforced concrete material is the most common material used in construction. This is due to its high
durability which makes concrete classified as one of the world building materials having the longest service
life. On the other hand, many research studies claimed that the application of concrete also has many durability
problems. This is even worse for extreme environments such as sea due to its high chloride ion concentration.
Environmental parameters such as temperature, humidity, and carbon dioxide concentration also have direct
effects on the deterioration of concrete structures. Nowadays, the Indonesian Government builds much
infrastructure which uses reinforced concrete as its main construction material. Concrete is chosen since its
constituents are abundant in Indonesia. Deterioration mechanisms of concrete structures can be divided into
the mechanism of concrete material deterioration and the mechanism of reinforcing bar corrosion. The
corrosion of reinforcing bar is the most dangerous mechanism and the most difficult one to control. Chloride
ions commonly coming from seawater become the main factor resulting in severe corrosion. This paper was
aimed to give suggestions for several durability-related parameters such as cement type, water-cement ratio,
concrete cover, etc. under Indonesian tropical climate.
1 INTRODUCTION
Indonesia, the world’s largest archipelagic state, has
a gigantic maritime domain of about six million
square kilometers. It consists of more than 17,000
islands with five largest islands being Sumatera, Java,
Kalimantan, Sulawesi, and Papua. Being located in
proximity to the equator line, Indonesia is influenced
mainly by the tropical rainforest climate. This climate
is typically denoted by its high temperature, heavy
rainfall, and high humidity. Its temperature is quite
stable over the year, ranging between 23 °C to 28 °C
spread from coastal plains to higher mountainous
areas. It shows only a small fluctuation from season
to season. Indonesia only has two main seasons,
namely wet or rainy season and dry season. Most
areas have their rainy season from September until
March, which reaches its peak in January and
February. Lowland areas have rainfall ranging
between 1800 to 3200 mm per year. These values
increase with the elevation of the area up to an
average of 6000 mm in some mountainous regions. In
a dry season, the rainfall decreases to 1800 mm
annually. This dry season occurs from April to
August with its driest peak occurring in July. The
relative humidity varies between 70% and 90% (Logt,
2016).
Nowadays, the Indonesian Government has more
focus on infrastructure development. It is achieved by
improving ports and maximizing inter-island
connectivity, and, in the end, it is hoped that
Indonesia becomes a “Global Maritime Axis”. The
priority of this infrastructure development plan is in
the infrastructure of the maritime sector. It is
considered to be a project of nationally strategic
importance given that Indonesia is the largest
archipelago in the world (Carruthers, 2016).
There are many seaports having been built
recently. Concrete is chosen to be their main
construction material since its constituents are
abundant in Indonesia. It is no wonder that reinforced
concrete becomes very popular. Its high durability
capacity makes concrete classified as one of the world
building materials having the longest service life. On
the other hand, many research studies claimed that the
application of concrete also has many durability
problems. This is even worse for extreme
environments such as sea due to its high chloride ion
Hilmy, M. and Prabowo, H.
Service Life Design for Infrastructure under Indonesian Environmental Exposure.
DOI: 10.5220/0008904000002481
In Proceedings of the Built Environment, Science and Technology International Conference (BEST ICON 2018), pages 133-138
ISBN: 978-989-758-414-5
Copyright
c
2022 by SCITEPRESS – Science and Technology Publications, Lda. All rights reserved
133