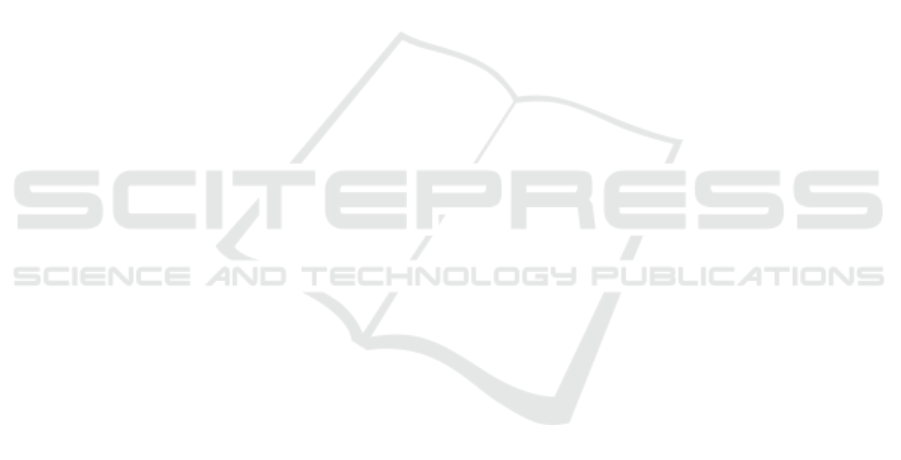
frequencies in many settings. In addition, the overall
time required for checking and post-processing the re-
sults of the new automatic workflow will in almost all
cases be significantly smaller than the time necessary
for fully manual annotation of plastids and stromu-
les. Instead of manually annotating several hundreds
of plastids and stromules by hand, usually only up
to 15% of the plastids and an even smaller fraction
of plastids with stromules per image requires manual
processing. This allows to extract large and repre-
sentative data sets much more efficiently than before
yielding a suitable basis for biological investigations.
5 CONCLUSIONS
The new image analysis workflow for the extraction
of stromule frequencies from wide field microscopy
images is capable of extracting reasonable quantita-
tive data suitable for biological investigations. Its per-
formance is comparable to those of human experts
while greatly reducing the time requirements. The
necessity for manual intervention is significantly re-
duced to a small fraction of the time that would be ne-
cessary for fully manual annotation. Thus, although
the overall workflow is not yet fully automatic and re-
lies on manual parameter tuning as well as on manual
validation and post-processing of results, our appro-
ach marks a significant improvement over the state-
of-the-art in stromule studies.
Future work will aim to further increase the de-
gree of automation and improve overall computatio-
nal efficiency and detection robustness, particularly
with regard to stromules. One possible direction will
be the investigation of machine learning techniques
for robust stromule identification particularly in ima-
ges with a high noise level and low quality.
ACKNOWLEDGEMENTS
This work has been supported by core funding of the
Martin Luther University Halle-Wittenberg, Saxony-
Anhalt, Germany, to B. M. and M. S.
REFERENCES
Basset, A., Boulanger, J., et al. (2015). Adaptive spot de-
tection with optimal scale selection in fluorescence
microscopy images. IEEE Trans. on Image Proc.,
24(11):4512–4527.
Bergeest, J.-P. and Rohr, K. (2012). Efficient globally opti-
mal segmentation of cells in fluorescence microscopy
images using level sets and convex energy functionals.
Medical Image Analysis, 16(7):1436 – 1444.
Buggenthin, F., Marr, C., et al. (2013). An automatic met-
hod for robust and fast cell detection in bright field
images from high-throughput microscopy. BMC Bi-
oinformatics, 14(1):297.
Chen, C., Wang, W., et al. (2013). A flexible and ro-
bust approach for segmenting cell nuclei from 2D mi-
croscopy images using supervised learning and tem-
plate matching. Cytometry Part A, 83A(5):495–507.
Erickson, J. L., Adlung, N., et al. (2017). The Xanthomo-
nas effector XopL uncovers the role of microtubules
in stromule extension and dynamics in nicotiana bent-
hamiana. The Plant Journal, 93(5):856–870.
Frangi, A. F., Niessen, W. J., et al. (1998). Multiscale vessel
enhancement filtering. In Medical Image Computing
and Computer-Assisted Intervention (MICCAI), pages
130–137. Springer Berlin Heidelberg.
Franke, L., Storbeck, B., et al. (2015). The ’MTB Cell
Counter’ a versatile tool for the semi-automated quan-
tification of sub-cellular phenotypes in fluorescence
microscopy images. A case study on plastids, nuclei
and peroxisomes. Journal of Endocytobiosis and Cell
Research, 26:31–42.
Fraz, M., Remagnino, P., et al. (2012). Blood vessel seg-
mentation methodologies in retinal images - a sur-
vey. Computer Methods and Programs in Biomedi-
cine, 108(1):407–433.
Gray, J. C., Hansen, M. R., et al. (2012). Plastid stromules
are induced by stress treatments acting through absci-
sic acid. The Plant Journal, 69(3):387–398.
Greß, O., M
¨
oller, B., et al. (2010). Scale-adaptive wavelet-
based particle detection in microscopy images. In
Meinzer, H.-P., Deserno, T. M., Handels, H., and Tolx-
dorff, T., editors, Bildverarbeitung f
¨
ur die Medizin,
Informatik Aktuell, pages 266–270, Berlin. Springer.
ISBN 978-3-642-11967-5.
Hanson, M. R. and Hines, K. M. (2018). Stromules:
probing formation and function. Plant Physiology,
176(1):128–137.
K
¨
ohler, R. H., Cao, J., et al. (1997). Exchange of protein
molecules through connections between higher plant
plastids. Science, 276(5321):2039–2042.
K
¨
ohler, R. H. and Hanson, M. R. (2000). Plastid tubules of
higher plants are tissue-specific and developmentally
regulated. Journal of Cell Science, 113:81–89.
Kraus, O. Z., Ba, J. L., and Frey, B. J. (2016). Classifying
and segmenting microscopy images with deep multi-
ple instance learning. Bioinformatics, 32(12):i52–i59.
Kumar, A. S., Park, E., et al. (2018). Stromule extension al-
ong microtubules coordinated with actin-mediated an-
choring guides perinuclear chloroplast movement du-
ring innate immunity. eLife, 7:e23625.
Marques, J. P., Schattat, M. H., et al. (2004). In vivo trans-
port of folded EGFP by the DeltapH/TAT-dependent
pathway in chloroplasts of Arabidopsis thaliana. Jour-
nal of Experimental Botany, 55(403):1697–1706.
Moghimirad, E., Rezatofighi, S. H., and Soltanian-Zadeh,
H. (2012). Retinal vessel segmentation using a multi-
Quantification of Stromule Frequencies in Microscope Images of Plastids Combining Ridge Detection and Geometric Criteria
47