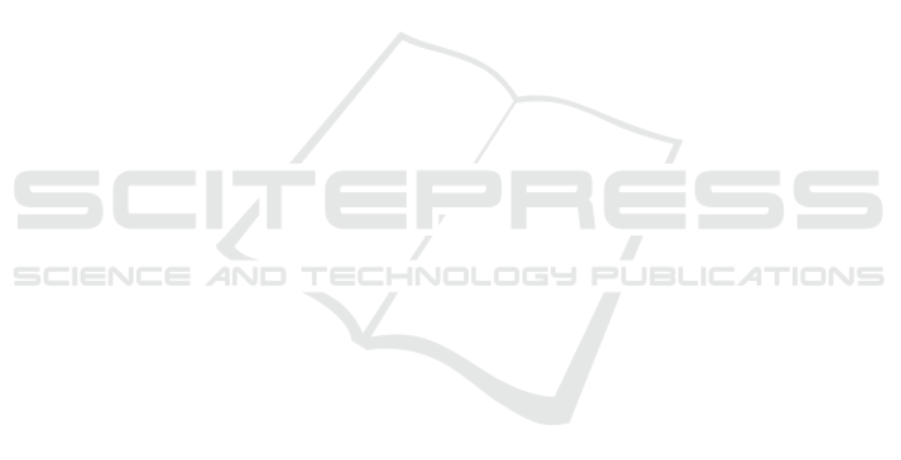
We derived the dependencies of the Mean
Glandular Dose changing breast anatomy and X-ray
beam; MGD decrease with both the increase of
compressed breast thickness and glandular
percentage. Important dependency is represented by
the HVL value (radiation beam quality), which can
lead to an overestimation of the glandular dose in case
of “harder” spectra. The rationale is to know the HVL
experimental value and to find the correct MGD value
referring to the specific radiation.
An important variable not yet permanently
defined in literature is the assessment of skin
thickness and composition. EU and US protocols
used, until 2016, respectively 5 mm and 4 mm thick
skin (now US protocol employs the 5 mm thick skin),
made by adipose tissue but, Huang et al. (Huang,
2008) found a different skin thickness in breast CT
investigations. A comparison between digital breast
phantoms with different skin showed of course
different MGD values. At low-energy investigations
skin 1.45 mm thick “shields” less glandular tissue,
respect to the 5 mm adipose skin, while for higher
energies shields more. This may be attributed to the
property of the thicker skin to “become a secondary
radiation source” at increasing energies, because of
the probability of the Compton effect (in the skin)
increases at the expense of the photoelectric effect
(Sarno, 2017). The choice of an appropriate model of
a digital breast phantom can be a critical aspect and
we reserve the right to continue investigating it.
In the last years, Digital Breast Tomosynthesis
(DBT) is spreading in clinics and represents an
evolution of mammography; this technique let the X-
ray tube to move in an arc over the compressed breast,
acquiring multiple images from different angles.
Images are then reconstructed by a computer forming
three-dimensional images. 3D techniques minimize
tissue overlaps that can hide cancers above the normal
overlapping.
We will improve our MC code implementing the
tomosynthesis set-up for dosimetry purposes.
To achieve the experimental verification of the
MC results, and improve the personalized dosimetry,
our efforts are focused on the creation of physical
phantoms with similar properties of the real breast,
like, of course geometry, but primarily X-ray
attenuation.
ACKNOWLEDGEMENTS
The presented work is part of the RADIOMA project
which is partially funded by "Fondazione Pisa",
Technological and Scientific Research Sector, Via
Pietro Toselli 29, Pisa (Italy). The authors would like
to thank Fondazione Pisa for giving the opportunity
to start this study.
REFERENCES
AAPM REPORT NO. 195. 2015. Monte Carlo Reference
Data Sets for Imaging Research.
Agostinelli, S. et al., 2003. GEANT4 - a simulation toolkit.
Nuclear Instruments and Methods in Physics Research
A 506 (2003) 250–303.
Boone, J.M., Fewell, T.R., Jennings, R.J., 1997.
Molybdenum, rhodium, and tungsten anode spectral
models using interpolating polynomials with
application to mammography. Med Phys. 1997
Dec;24(12):1863-74.
Boone, J.M., 1999. Glandular Breast Dose for
Monoenergetic and High-Energy X-ray Beams: Monte
Carlo Assessment. Radiology. 1999 Oct;213(1):23-37.
Boone, J.M., 2002. Normalized glandular dose (DgN)
coefficients for arbitrary x-ray spectra in
mammography: Computer-fit values of Monte Carlo
derived data. Med Phys. 2002 May;29(5):869-75.
Dance, D.R., 1990. Monte Carlo Calculation of conversion
factor for the estimation of mean glandular breast dose.
Phys Med Biol. 1990 Sep;35(9):1211-9.
Dance, D.R., Sechopoulos, I., 2016. Dosimetry in x-ray
based breast imaging. Phys Med Biol. 2016 Oct
7;61(19):R271-R304.
Gholamkar, L., Mowlavi, L.,A., Sadeghi, M., Athari, M.,
2016. Assessment of Mean Glandular Dose in
Mammography System with Different Anode-Filter
Combinations Using MCNP Code. Iran J Radiol. 2016
October; 13(4):e36484.
Hernandez, A.M., Seibert, J.A., Nosratieh. A,, Boone, J.M.,
2017. Generation and analysis of clinically relevant
breast imaging x-ray spectra. Med Phys. 2017
Jun;44(6):2148-2160.
Huang, S., Boone, J.M., Yang, K., Kwan, A.L.C., Packard,
N.J., 2008. The effect of skin thickness determined
using breast CT on mammographic dosimetry. Med
Phys. 2008 Apr;35(4):1199-206.
Hubbell, J.H., Seltzer, S.M., 1995. Tables of X-Ray Mass
Attenuation Coefficients and Mass Energy-Absorption
Coefficients from 1 keV to 20 MeV for Elements Z = 1
to 92 and 48 Additional Substances of Dosimetric
Interest. NISTIR 5632.
Massera, R.T., Tomal, A., 2018. Skin models and their
impact on mean glandular dose in mammography.
Physica Medica Volume 51, July 2018, Pages 38-47.
Nigapruke, K., Puwanich, P., Phaisangittisakul, N.,
Youngdee, W., 2010. Monte Carlo Simulation of
Average Glandular Dose and an Investigation of
Influencing Factors. Journal of Radiation Research,
2010 51(4), 441–448.
Nosratieh, A., Hernandez, A,. Shen, S.Z., Yaffe, M.J.,
Seibert, J.A., Boone, J.M., 2015. Mean glandular dose
coefficients (DgN) for x-ray spectra used in
BIOINFORMATICS 2019 - 10th International Conference on Bioinformatics Models, Methods and Algorithms
248