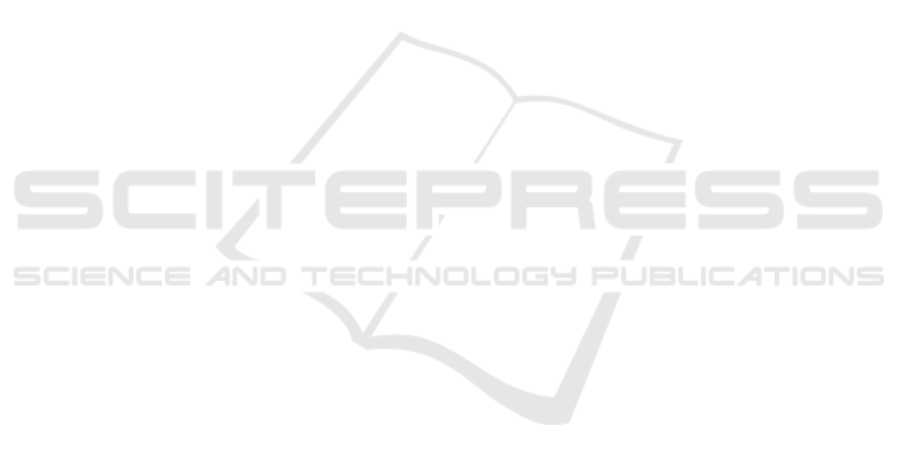
ACKNOWLEDGEMENTS
We would like to acknowledge the financial support
obtained from North Portugal Regional Operational
Programme (NORTE 2020), Portugal 2020 and the
European Regional Development Fund (ERDF) from
European Union through the project Symbiotic tech-
nology for societal efficiency gains: Deus ex Machina
(DEM), NORTE-01-0145-FEDER-000026.
For supporting with the necessary material and
guidance we would also like to acknowledge Lab-
orat
´
orio de
´
Optica, Lasers e Sistemas, a contract-
research laboratory within the Physics Department of
FCUL (University of Lisbon).
It is also important to acknowledge Ricardo
Peixoto, Jo
˜
ao Gonc¸alves, Sim
˜
ao Felgueiras and Jo
˜
ao
Oliveira, the Fraunhofer AICOS team members re-
sponsible for assembling and developing the proto-
type.
REFERENCES
Calandra, D. M., Di Martino, S., Riccio, D., and Visconti,
A. (2017). Smartphone based pupillometry: An em-
pirical evaluation of accuracy and safety. In Battiato,
S., Gallo, G., Schettini, R., and Stanco, F., editors,
Image Analysis and Processing - ICIAP 2017, pages
433–443, Cham. Springer International Publishing.
Capit
´
an-Vallvey, L. F. and Palma, A. J. (2011). Recent de-
velopments in handheld and portable optosensing—a
review. Analytica Chimica Acta, 696(1):27 – 46.
Chen, H. (2005). Method for manufacturing a triple wave-
lengths white led.
DeHoog, E. and Schwiegerling, J. (2009). Fundus cam-
era systems: a comparative analysis. Appl Opt,
48(2):221–228. 19137032[pmid].
Desmet, K. D., Paz, D. A., Corry, J. J., Eells, J. T., Wong-
Riley, M. T., Henry, M. M., Buchmann, E. V., Con-
nelly, M. P., Dovi, J. V., Liang, H. L., Henshel,
D. S., Yeager, R. L., Millsap, D. S., Lim, J., Gould,
L. J., Das, R., Jett, M., Hodgson, B. D., Margo-
lis, D., and Whelan, H. T. (2006). Clinical and ex-
perimental applications of nir-led photobiomodula-
tion. Photomedicine and Laser Surgery, 24(2):121–
128. PMID: 16706690.
Dyble, M., Narendran, N., Bierman, A., Klein, T., Dy-
ble, M., Narendran, N., Bierman, A., and Klein, T.
(2005). Impact of Dimming White LEDs : Chromatic-
ity Shifts Due to Different Dimming Methods.
Eells, J. T., Wong-Riley, M. T., VerHoeve, J., Henry, M.,
Buchman, E. V., Kane, M. P., Gould, L. J., Das, R.,
Jett, M., Hodgson, B. D., Margolis, D., and Whelan,
H. T. (2004). Mitochondrial signal transduction in ac-
celerated wound and retinal healing by near-infrared
light therapy. Mitochondrion, 4(5):559 – 567. Mito-
chondrial Medicine - Developing the Scientific Basis
to Medical Management of Mitochondrial Disease.
Feder, R. S., Olsen, T. W., Prum, B. E. J., Summers, C. G.,
Olson, R. J., Williams, R. D., and Musch, D. C.
(2016). Comprehensive adult medical eye evaluation
preferred practice pattern guidelines. Ophthalmology,
123(1):P209–P236.
Hammer, M. and Schweitzer, D. (2002). Quantitative reflec-
tion spectroscopy at the human ocular fundus. Physics
in Medicine & Biology, 47(2):179.
Hong, S. C., Wynn-Williams, G., and Wilson, G. (2017).
Safety of iphone retinal photography. Journal of
Medical Engineering & Technology, 41(3):165–169.
PMID: 27924670.
ISO 10940 (2009). Ophthalmic instruments - Fundus cam-
eras. Standard, International Organization for Stan-
dardization, Geneva, CH.
ISO 15004-2 (2007). Ophthalmic instruments - Fundamen-
tal requirements and test methods.Part 2: Light Haz-
ard Protection. Standard, International Organization
for Standardization, Geneva, CH.
Kelbsch, C., Maeda, F., Strasser, T., Blumenstock, G.,
Wilhelm, B., Wilhelm, H., and Peters, T. (2016).
Pupillary responses driven by iprgcs and classical
photoreceptors are impaired in glaucoma. Graefe’s
Archive for Clinical and Experimental Ophthalmol-
ogy, 254(7):1361–1370.
Malinen, J., K
¨
ans
¨
akoski, M., Rikola, R., and Eddison, C. G.
(1998). Led-based nir spectrometer module for hand-
held and process analyser applications. Sensors and
Actuators B: Chemical, 51(1):220 – 226.
Patrick, D. and Fardo, S. (2000). Industrial electronics: De-
vices and systems.
Preece, S. J. and Claridge, E. (2002). Monte carlo mod-
elling of the spectral reflectance of the human eye.
Physics in Medicine & Biology, 47(16):2863.
Rahman, M. Q., Rotchford, A. P., and Ramaesh, K. (2013).
Catatrac: a novel red light-emitting diode device for
screening cataracts in the developing world. Eye
(Lond), 27(1):37–41. 23099916[pmid].
Ready, J. F. (1971). Chapter 2 - measurement techniques.
In Ready, J. F., editor, Effects of High-Power Laser
Radiation, pages 33 – 65. Academic Press.
Rothem, E. Measuring led power and irradiance with cali-
brated photodiodes. Technical report, Ophir.
Rukmini, A. V., Milea, D., Baskaran, M., How, A. C., Per-
era, S. A., Aung, T., and Gooley, J. J. (2015). Pupil-
lary responses to high-irradiance blue light correlate
with glaucoma severity. Ophthalmology, 122(9):1777
– 1785.
Saltmarche, A. E., Naeser, M. A., Ho, K. F., Hamblin,
M. R., and Lim, L. (2017). Significant improvement
in cognition in mild to moderately severe dementia
cases treated with transcranial plus intranasal photo-
biomodulation: Case series report. Photomed Laser
Surg, 35(8):432–441. 28186867[pmid].
Schubert, E. (2018). Light-Emitting Diodes (3rd Edition).
Senior, J. (1986). Optical Fiber Communications: Princi-
ples and Practice. Prentice Hall International (UK)
Ltd., Hertfordshire, UK, UK.
Light Hazard Measurement on an Ophthalmic Instrument
97