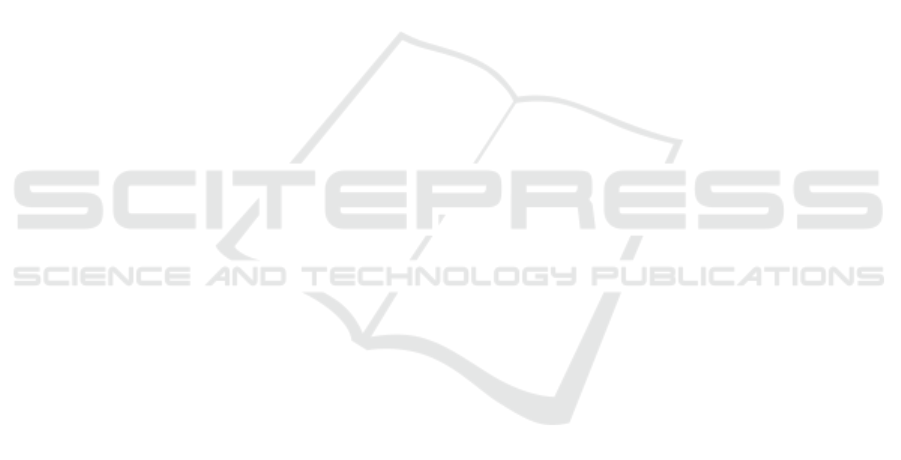
used within traumatology, so their validity when rep-
resenting these microstructures remain in no man’s
land and can not be exploited by specialists. This
study aims to lay the foundations for the virtual gener-
ation of bone tissue not only from a geometric point of
view, but also from a medical point of view. The study
of the segmentation of the bone model, how it should
be generated in order to evaluate and compare it later,
and the establishment of a series of limits serve as a
starting point for achieving this ambitious objective.
For the future it would be interesting to generate the
different microstructures that make up the bone tis-
sue taking into account all those elements that are
scientifically relevant such as the different measures
used. What other types of structural parameters could
be included to make the tissue generated more useful
within the different fields of study?
ACKNOWLEDGEMENTS
This work has been subsidized by the Ministry of
Economy and Competitiveness and the European
Union (via ERDF funds) through the research project.
DPI2015-65123-R.
REFERENCES
Akkiraju, N., Edelsbrunner, H., Facello, M., Fu, P., Mucke,
E., and Varela, C. (1995). Alpha shapes: definition
and software. IEEE Transactions on Information The-
ory, 63(1):66–70.
Boykov, Y. and Funka-Lea, G. (2006). Graph cuts and effi-
cient n-d image segmentation.
Britz, H. M., Thomas, C. D. L., Clement, J. G., and Cooper,
D. M. (2009). The relation of femoral osteon geome-
try to age, sex, height and weight. Bone, 45(1):77–83.
Cardoso, L., Fritton, S. P., Gailani, G., Benalla, M., and
Cowin, S. C. (2013). Advances in assessment of bone
porosity, permeability and interstitial fluid flow. Jour-
nal of Biomechanics, 46(2):253–265.
Demirtas, A., Curran, E., and Ural, A. (2016). Assessment
of the effect of reduced compositional heterogeneity
on fracture resistance of human cortical bone using
finite element modeling. Bone, 91:92–101.
Descoteaux, M., Audette, M., Chinzei, K., and Siddiqi, K.
(2006). Bone enhancement filtering: Application to
sinus bone segmentation and simulation of pituitary
surgery. Computer Aided Surgery, 11(5):247–255.
Doblar
´
e, M., Garc
´
ıa, J., and G
´
omez, M. (2004). Modelling
bone tissue fracture and healing: a review. Engineer-
ing Fracture Mechanics, 71(13-14):1809–1840.
Edelsbrunner, H., Kirkpatrick, D., and Seidel, R. (1983).
On the shape of a set of points in the plane. IEEE
Transactions on Information Theory, 29(4):551–559.
Fan, J., Zeng, G., Body, M., and Hacid, M.-S. (2005).
Seeded region growing: an extensive and comparative
study. Pattern Recognition Letters, 26(8):1139–1156.
Gao, X., Li, S., Adel-Wahab, A., and Silberschmidt, V.
(2013). Effect of random microstructure on crack
propagation in cortical bone tissue under dynamic
loading. Journal of Physics: Conference Series,
451:012033.
Hennig, C., Thomas, C. D. L., Clement, J. G., and Cooper,
D. M. L. (2015). Does 3d orientation account for vari-
ation in osteon morphology assessed by 2d histology?
Journal of Anatomy, 227(4):497–505.
Justice, R., Stokely, E., Strobel, J., Ideker, R., and Smith, W.
(1997). Medical image segmentation using 3d seeded
region grow-ing. Medical Imaging 1997: Image Pro-
cessing, 3034:900–910.
Lin, Y. and Xu, S. (2010). AFM analysis of the lacunar-
canalicular network in demineralized compact bone.
Journal of Microscopy, 241(3):291–302.
Najafi, A. R., Arshi, A., Eslami, M., Fariborz, S., and
Moeinzadeh, M. (2007). Micromechanics fracture in
osteonal cortical bone: A study of the interactions
between microcrack propagation, microstructure and
the material properties. Journal of Biomechanics,
40(12):2788–2795.
Nobakhti, S., Limbert, G., and Thurner, P. J. (2014). Ce-
ment lines and interlamellar areas in compact bone
as strain amplifiers – contributors to elasticity, frac-
ture toughness and mechanotransduction. Journal of
the Mechanical Behavior of Biomedical Materials,
29:235–251.
Paulano, F., Jim
´
enez, J. J., and Pulido, R. (2014). 3d seg-
mentation and labeling of fractured bone from CT im-
ages. The Visual Computer, 30(6-8):939–948.
Paulano-Godino, F., Jim
´
enez-P
´
erez, J. R., and Jim
´
enez-
Delgado, J. J. (2017). Issues on the simulation of geo-
metric fractures of bone models. In VipIMAGE 2017,
pages 467–475. Springer International Publishing.
P
´
erez, F. D., Jim
´
enez, J. J., and Jim
´
enez, J. R. (2018).
Blast Features and Requirements for Fracturing Os-
seous Models. In Garc
´
ıa-Fern
´
andez, I. and Ure
˜
na,
C., editors, Spanish Computer Graphics Conference
(CEIG). The Eurographics Association.
Sabet, F. A., Najafi, A. R., Hamed, E., and Jasiuk, I.
(2015). Modelling of bone fracture and strength at
different length scales: a review. Interface Focus,
6(1):20150055.
Tong, X., Burton, I. S., Isaksson, H., Jurvelin, J. S., and
Kr
¨
oger, H. (2015). Cortical bone histomorphome-
try in male femoral neck: The investigation of age-
association and regional differences. Calcified Tissue
International, 96(4):295–306.
T
¨
ornquist, E. (2017). Segmentation, analysis, and mod-
elling of microstructure in cortical bone, based on x-
ray microtomography. Student Paper.
Ural, A. (2011). Cohesive modeling of bone fracture at mul-
tiple scales. Procedia Engineering, 10:2827–2832.
Towards the Modelling of Osseous Tissue
345