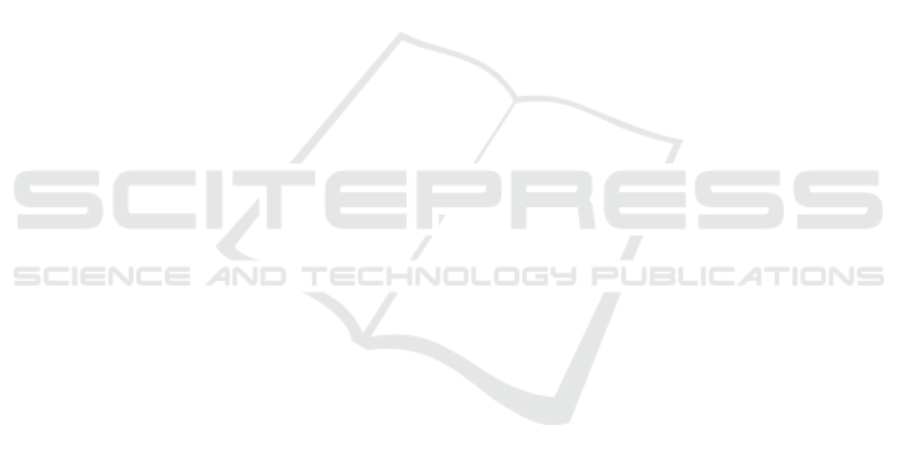
deformation inside the US view, leading to improve
the learner immersion sense.
Next step will aim to further improve the visual
rendering of the US image by adding others arti-
facts, and add more complex and precise displace-
ment force, based on physically-based simulations.
ACKNOWLEDGMENTS
This work was supported by French state funds
managed by the ANR within the IDEFI-SAMSEI
(Strat
´
egies d’Apprentissage des M
´
etiers de Sant
´
e en
Environnement Immersif) project. We thank rheuma-
tologist Dr.Coury-Lucas for her valuable feedback,
and E. Galin and J-C. Iehl for their help.
REFERENCES
Abolhassani, N., Patel, R., and Moallem, M. (2007). Needle
insertion into soft tissue: A survey. Medical engineer-
ing & physics, 29(4):413–431.
Bamber, J. and Dickinson, R. (1980). Ultrasonic B-scanning:
a computer simulation. Physics in Medicine & Biology,
25(3):463.
Burger, B., Bettinghausen, S., Radle, M., and Hesser, J.
(2013). Real-time gpu-based ultrasound simulation us-
ing deformable mesh models. IEEE transactions on
medical imaging, 32(3):609–618.
Crouch, J. R., Schneider, C. M., Wainer, J., and Okamura,
A. M. (2005). A velocity-dependent model for needle
insertion in soft tissue. In International Conference on
Medical Image Computing and Computer-Assisted In-
tervention, pages 624–632. Springer.
DiMaio, S. P. and Salcudean, S. E. (2003). Needle inser-
tion modeling and simulation. IEEE Transactions on
robotics and automation, 19(5):864–875.
Duriez, C., Gu
´
ebert, C., Marchal, M., Cotin, S., and Grisoni,
L. (2009). Interactive simulation of flexible nee-
dle insertions based on constraint models. In In-
ternational Conference on Medical Image Computing
and Computer-Assisted Intervention, pages 291–299.
Springer.
Flack, B., Makhinya, M., and Goksel, O. (2016). Model-
based compensation of tissue deformation during data
acquisition for interpolative ultrasound simulation. In
Biomedical Imaging (ISBI), 2016 IEEE 13th Interna-
tional Symposium on, pages 502–505. IEEE.
Gao, D., Lei, Y., and Yao, B. (2013). Analysis of dynamic tis-
sue deformation during needle insertion into soft tissue.
IFAC Proceedings Volumes, 46(5):684–691.
Goksel, O. and Salcudean, S. E. (2009). B-mode ultrasound
image simulation in deformable 3-D medium. IEEE
transactions on medical imaging, 28(11):1657–1669.
Harrison, P. (2001). A non-hierarchical procedure for re-
synthesis of complex textures. WSCG ’2001: Confer-
ence proceedings: University of West Bohemia, Plzen,
Czech Republic, pages 190–197.
Heer, I., Middendorf, K., M
¨
uller-Egloff, S., Dugas, M., and
Strauss, A. (2004). Ultrasound training: the virtual
patient. Ultrasound in Obstetrics and Gynecology,
24(4):440–444.
Jensen, J. A. (1991). A model for the propagation and scat-
tering of ultrasound in tissue. The Journal of the Acous-
tical Society of America, 89(1):182–190.
Law, Y. C., Ullrich, S., Knott, T., Kuhlen, T., and Weg, S.
(2011). Ultrasound image simulation with gpu-based
ray tracing. Virtuelle und Erweiterte Realit
¨
at, 8:183–
194.
Magee, D. and Kessel, D. (2005). A computer based simula-
tor for ultrasound guided needle insertion procedures.
IEE International Conference on Visual Information
Engineering (VIE 2005), pages 301–308.
Magee, D., Zhu, Y., Ratnalingam, R., Gardner, P., and Kessel,
D. (2007). An augmented reality simulator for ultra-
sound guided needle placement training. Medical & bi-
ological engineering & computing, 45(10):957–967.
Marion, A. and Vray, D. (2009). Toward a real-time simula-
tion of ultrasound image sequences based on a 3-d set
of moving scatterers. IEEE transactions on ultrason-
ics, ferroelectrics, and frequency control, 56(10):2167–
2179.
Mattausch, O. and Goksel, O. (2016). Monte-carlo ray-
tracing for realistic interactive ultrasound simulation. In
Proceedings of the Eurographics Workshop on Visual
Computing for Biology and Medicine, pages 173–181.
Eurographics Association.
McReynolds, T. and Blythe, D. (2005). Advanced graphics
programming using OpenGL. Elsevier.
Ni, D., Chan, W. Y., Qin, J., Chui, Y.-P., Qu, I., Ho, S. S.,
and Heng, P.-A. (2011). A virtual reality simulator
for ultrasound-guided biopsy training. IEEE Computer
Graphics and Applications, 31(2):36–48.
Okamura, A. M., Simone, C., and O’leary, M. D. (2004).
Force modeling for needle insertion into soft tis-
sue. IEEE transactions on biomedical engineering,
51(10):1707–1716.
Panozzo, D., Puppo, E., and Rocca, L. (2010). Effi-
cient multi-scale curvature and crease estimation. Pro-
ceedings of Computer Graphics, Computer Vision and
Mathematics (Brno, Czech Rapubic, 1(6).
Takabi, B. and Tai, B. L. (2017). A review of cutting me-
chanics and modeling techniques for biological materi-
als. Medical engineering & physics, 45:1–14.
van Gerwen, D. J., Dankelman, J., and van den Dobbelsteen,
J. J. (2012). Needle–tissue interaction forces–a survey
of experimental data. Medical engineering & physics,
34(6):665–680.
Vidal, F. P., John, N. W., Healey, A. E., and Gould, D. A.
(2008). Simulation of ultrasound guided needle punc-
ture using patient specific data with 3D textures and vol-
ume haptics. Computer Animation and Virtual Worlds,
19(2):111–127.
Yang, C., Xie, Y., Liu, S., and Sun, D. (2018). Force
modeling, identification, and feedback control of robot-
assisted needle insertion: a survey of the literature. Sen-
sors, 18(2):561.
Zilles, C. B. and Salisbury, J. K. (1995). A constraint-based
god-object method for haptic display. In Intelligent
Robots and Systems 95.’Human Robot Interaction and
Cooperative Robots’, Proceedings. 1995 IEEE/RSJ In-
ternational Conference on, volume 3, pages 146–151.
IEEE.
GRAPP 2020 - 15th International Conference on Computer Graphics Theory and Applications
246