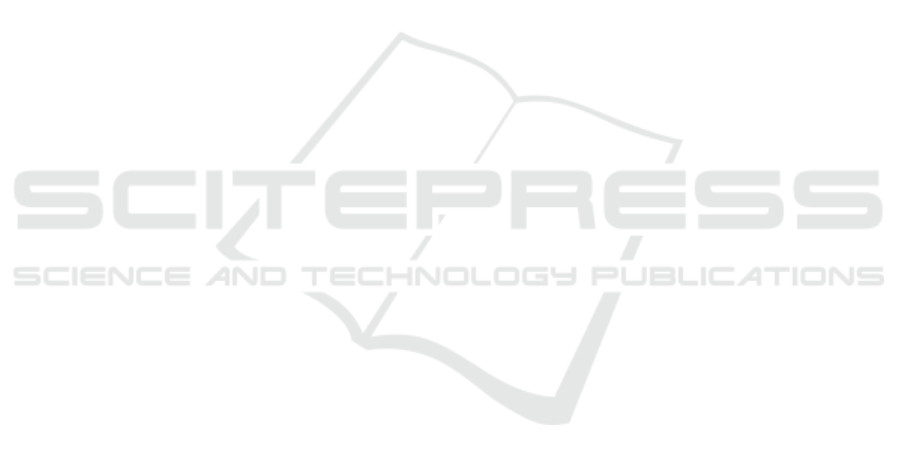
Di Giulio, M. (2017). Some pungent arguments against
the physico-chemical theories of the origin of the ge-
netic code and corroborating the coevolution theory. J
Theor Biol, 414:1–4.
Dudkiewicz, A., Mackiewicz, P., Nowicka, A., Kowalezuk,
M., Mackiewicz, D., Polak, N., Smolarczyk, K., Ba-
naszak, J., Dudek, M. R., and Cebrat, S. (2005).
Correspondence between mutation and selection pres-
sure and the genetic code degeneracy in the gene
evolution. Future Generation Computer Systems,
21(7):1033–1039.
Epstein, C. J. (1966). Role of the amino-acid ”code” and of
selection for conformation in the evolution of proteins.
Nature, 210(5031):25–8.
Freeland, S. J. and Hurst, L. D. (1998). The genetic code is
one in a million. J Mol Evol, 47(3):238–248.
Freeland, S. J., Knight, R. D., Landweber, L. F., and Hurst,
L. D. (2000). Early fixation of an optimal genetic
code. Mol Biol Evol, 17(4):511–8.
Freeland, S. J., Wu, T., and Keulmann, N. (2003). The
case for an error minimizing standard genetic code.
Origins of Life and Evolution of the Biosphere, 33(4-
5):457–477.
Gomes, A. C., Miranda, I., Silva, R. M., Moura, G. R.,
Thomas, B., Akoulitchev, A., and Santos, M. A.
(2007). A genetic code alteration generates a pro-
teome of high diversity in the human pathogen Can-
dida albicans. Genome Biol, 8(10):R206.
Goodarzi, H., Najafabadi, H. S., Hassani, K., Nejad, H. A.,
and Torabi, N. (2005). On the optimality of the ge-
netic code, with the consideration of coevolution the-
ory by comparison of prominent cost measure matri-
ces. J Theor Biol, 235(3):318–25.
Haig, D. and Hurst, L. D. (1991). A quantitative measure
of error minimization in the genetic-code. J Mol Evol,
33(5):412–417.
Heaphy, S. M., Mariotti, M., Gladyshev, V. N., Atkins, J. F.,
and Baranov, P. V. (2016). Novel ciliate genetic code
variants including the reassignment of all three stop
codons to sense codons in Condylostoma magnum.
Mol Biol Evol, 33:2885–2889.
Hoffman, D. C., Anderson, R. C., DuBois, M. L.,
and Prescott, D. M. (1995). Macronuclear gene-
sized molecules of hypotrichs. Nucleic Acids Res,
23:1279–1283.
Janouskovec, J., Sobotka, R., Lai, D.-H., Flegontov, P.,
Kon
´
ık, P., Komenda, J., Ali, S., Pr
´
a
ˇ
sil, O., Pain, A.,
Oborn
´
ık, M., Luke
ˇ
s, J., and Keeling, P. J. (2013). Split
photosystem protein, linear-mapping topology, and
growth of structural complexity in the plastid genome
of Chromera velia. Molecular Biology and Evolution,
30(11):2447–2462.
Kurnaz, M. L., Bilgin, T., and Kurnaz, I. A. (2010). Certain
non-standard coding tables appear to be more robust
to error than the standard genetic code. J Mol Evol,
70(1):13–28.
Mackiewicz, P., Biecek, P., Mackiewicz, D., Kiraga, J.,
Baczkowski, K., Sobczynski, M., and Cebrat, S.
(2008). Optimisation of asymmetric mutational pres-
sure and selection pressure around the universal ge-
netic code. Computational Science - Iccs 2008, Pt 3,
5103:100–109.
Massey, S. E. (2008). A neutral origin for error minimiza-
tion in the genetic code. J Mol Evol, 67(5):510–516.
Massey, S. E. and Garey, J. R. (2007). A comparative ge-
nomics analysis of codon reassignments reveals a link
with mitochondrial proteome size and a mechanism
of genetic code change via suppressor tRNAs. J Mol
Evol, 64(4):399–410.
McCutcheon, J. P., McDonald, B. R., and Moran, N. A.
(2009). Origin of an alternative genetic code in the
extremely small and GC-rich genome of a bacterial
symbiont. PLoS Genetics, 5(7):e1000565.
Morgens, D. W. and Cavalcanti, A. R. (2013). An alterna-
tive look at code evolution: using non-canonical codes
to evaluate adaptive and historic models for the origin
of the genetic code. J Mol Evol, 76(1-2):71–80.
Nakamura, Y., Gojobori, T., and Ikemura, T. (2000). Codon
usage tabulated from the international DNA sequence
databases: status for the year 2000. Nucleic Acids Res,
28:292.
Novozhilov, A. S., Wolf, Y. I., and Koonin, E. V. (2007).
Evolution of the genetic code: partial optimization of
a random code for robustness to translation error in a
rugged fitness landscape. Biol Direct, 2:24.
Perseke, M., Hetmank, J., Bernt, M., Stadler, P. F., Schlegel,
M., and Bernhard, D. (2011). he enigmatic mito-
chondrial genome of Rhabdopleura compacta (Pter-
obranchia) reveals insights into selection of an effi-
cient tRNA system and supports monophyly of Am-
bulacraria. BMC Evolutionary Biology, 11:134.
P
´
anek, T.,
ˇ
Zihala, D., Sokol, M., Derelle, R., Klime
ˇ
s, V.,
Hradilov
´
a, M., Zadrob
´
ılkov
´
a, E., Susko, E., Roger,
A. J.,
ˇ
Cepi
ˇ
cka, I., and Eli
´
a
ˇ
s, M. (2017). Nuclear ge-
netic codes with a different meaning of the UAG and
the UAA codon. BMC Biology, 15:8.
Sanchez-Silva, R., Villalobo, E., Morin, L., and Torres, A.
(2003). A new noncanonical nuclear genetic code:
Translation of UAA into glutamate. Current Biology,
13(5):442–447.
Santos, J. and Monteagudo, A. (2010). Study of the genetic
code adaptability by means of a genetic algorithm. J
Theor Biol, 264(3):854–865.
Santos, J. and Monteagudo, A. (2011). Simulated evolution
applied to study the genetic code optimality using a
model of codon reassignments. BMC Bioinformatics,
12.
Santos, J. and Monteagudo,
´
A. (2017). Inclusion of the fit-
ness sharing technique in an evolutionary algorithm
to analyze the fitness landscape of the genetic code
adaptability. BMC Bioinformatics, 18(1):195.
Santos, M. A., Cheesman, C., Costa, V., Moradas-Ferreira,
P., and Tuite, M. F. (1999). Selective advantages cre-
ated by codon ambiguity allowed for the evolution of
an alternative genetic code in Candida spp. Molecular
Microbiology, 31:937–947.
Santos, M. A., Keith, G., and Tuite, M. F. (1993). Non-
standard translational events in Candida albicans me-
diated by an unusual seryl-tRNA with a 5’-CAG-3’
(leucine) anticodon. The EMBO Journal, 12:607–616.
BIOINFORMATICS 2020 - 11th International Conference on Bioinformatics Models, Methods and Algorithms
50