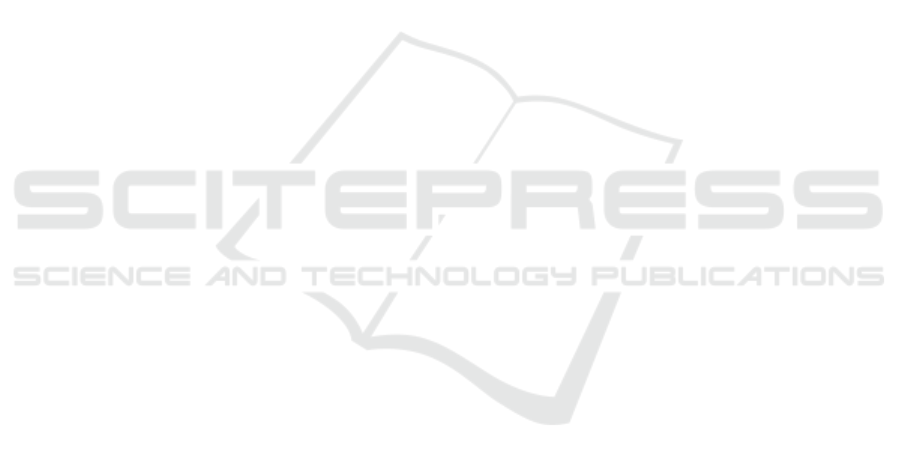
5.2 Sympathetic Sedation Time
In this verification, the sympathetic sedation time was
calculated using only the RRV of the electrocardio-
gram as an index, but RRV is affected by the respira-
tory cycle as well as the sympathetic nerves. The re-
sults must be verified from various angles using other
sympathetic nerve indexes, such as pupil diameter,
electro dermal activity, etc. In addition, the sym-
pathetic nerves were sedated when RRV after two-
back exceeded 60% of the maximum value for the
first time, but the threshold value of 60% is necessary
for comparison with various threshold values of other
sympathetic nerve indexes.
5.3 Stressor
In this study, the two-back task was selected as a stres-
sor that secretes cortisol, but this task leads to a low
amount of cortisol secretion (Henckens et al., 2011).
Since the proposed method is intended to measure the
decrease in the negative feedback mechanism of cor-
tisol, the stressor needs to cause the secretion of corti-
sol. For example, the Trier Social Stress Test (TSST)
results in the secretion of cortisol, but the process is
very long and the second presentation shows acclima-
tization and significantly reduced cortisol secretion
(Yao et al., 2016; Dhabhar et al., 1997; W
¨
ust et al.,
2005; Schommer et al., 2003). We need to design a
cortisol-secreting stressor that can be presented in a
short period of time and with little familiarity.
6 CONCLUSION
In this study, we propose chronic stress estimation
through sympathetic sedation time measurements as
a non-invasive, fast, and highly accurate method. In
this method, a stressor is applied to an user, the time
until the sympathetic nerve activity subsides is mea-
sured, and the chronic stress state is estimated from
the length of time.
We recruited nine participants to this study. As
a result, we confirmed that the sympathetic sedation
time tended to be longer in the chronic stress group
than in the healthy group by performing fast respira-
tory control after stressor loading. Moreover, we be-
lieve that the following improvements could make the
demonstration of feasibility more reliable.
1. Multifaceted verification of whether the sympa-
thetic nerve has sedated.
2. Optimal stressor design for sympathetic sedation
time measurement.
After demonstrating the feasibility of chronic
stress estimation by measuring sympathetic sedation
time through the above improvements, we aim to de-
sign a wearable device that integrates a sensor for
measuring sympathetic sedation time as well as a
stressor presentation device. We believe that with the
development of such devices, anyone will be able to
identify their chronic stress state on a daily basis and
prevent mental illnesses, such as major depression.
REFERENCES
Carreiras, C., Alves, A. P., et al. (2015–). BioSPPy: Biosig-
nal processing in Python. [Online; accessed 2018-8-
2].
Clinic, M. (2020). Mitsuoka clinic. https://www.mitsuoka-
clinic.com/depression2.htm.
Cohen, S., Kamarck, T., and Mermelstein, R. (1983). A
global measure of perceived stress. Journal of health
and social behavior, pages 385–396.
Contoreggi, C.(2015). Corticotropin releasing hormone and
imaging, rethinking the stress axis. Nuclear medicine
and biology, 42(4):323–339.
Dhabhar, F. S., McEwen, B. S., and Spencer, R. L.(1997).
Adaptation to prolonged or repeated stress–
comparison between rat strains showing intrinsic
differences in reactivity to acute stress. Neuroen-
docrinology, 65(5):360–368.
Dogan, E., Sander, C., Wagner, X., Hegerl, U., and Kohls,
E. (2017). Smartphone-based monitoring of objective
and subjective data in affective disorders: Where are
we and where are we going? systematic review. Jour-
nal of Medical Internet Research, 19.
Fink, G.(2010). Encyclopedia of STRESS, 2nd Ed.
Maruzen.
Habib, K. E., Weld, K. P., Rice, K. C., Pushkas, J., Cham-
poux, M., Listwak, S., Webster, E. L., Atkinson, A. J.,
Schulkin, J., Contoreggi, C., et al.(2000). Oral ad-
ministration of a corticotropin-releasing hormone re-
ceptor antagonist significantly attenuates behavioral,
neuroendocrine, and autonomic responses to stress in
primates. Proceedings of the National Academy of
Sciences, 97(11):6079–6084.
Hashimoto, T. et al.(2017). Expression analyses of stress-
related factors in the brain of the single prolonged
stress rats. Human developmental research CODER
annual Report, 31:105–113.
Hellhammer, D. H., W
¨
ust, S., and Kudielka, B. M.(2009).
Salivary cortisol as a biomarker in stress research.
Psychoneuroendocrinology, 34(2):163–171.
Henckens, M. J., van Wingen, G. A., Jo
¨
els, M., and
Fern
´
andez, G.(2011). Time-dependent corticosteroid
modulation of prefrontal working memory process-
ing. Proceedings of the National Academy of Sciences,
108(14):5801–5806.
Heuser, I., Yassouridis, A., and Holsboer, F. (1994). The
combined dexamethasone/crh test: a refined labora-
Estimation of Chronic Stress by Measuring Sympathetic Sedation Time
297