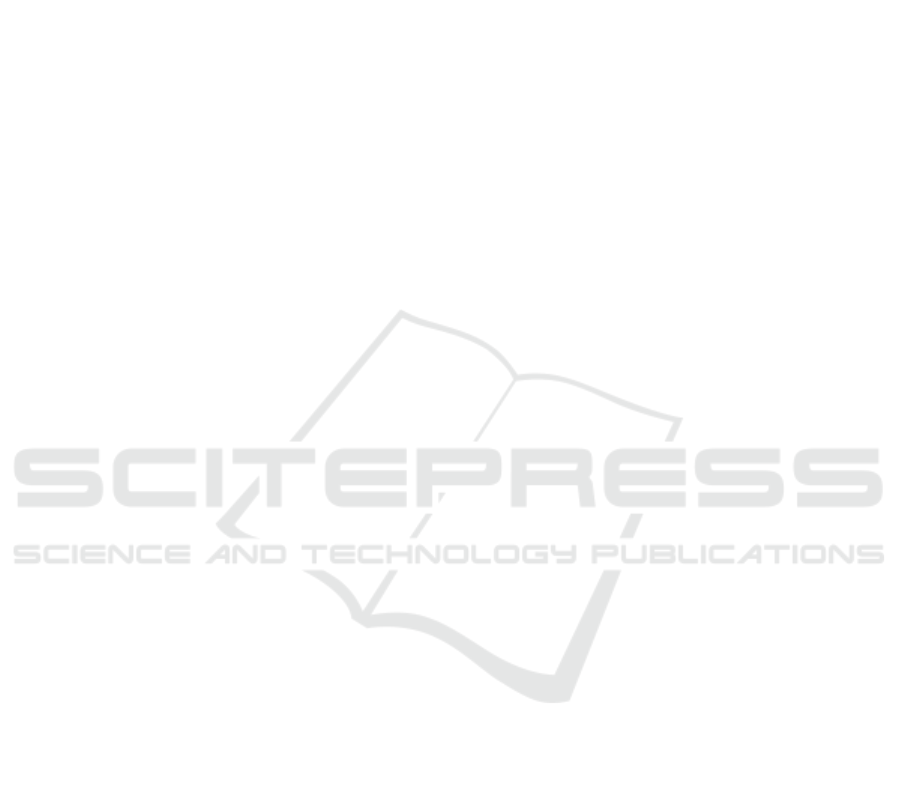
As expected, the grey value increased from the
entry to the exit, indicating a reduction of the particle
concentration throughout the microchannels.
Difference in particle size does not seem to affect the
clearance process since the results were similar using
both of the analogue fluids. On the other hand, the
fluid flow affects the efficiency of the microchannel.
For first manufacture process it was possible to obtain
high grey values corresponding to a reduction of the
particle concentration. The grey values were also near
the value obtained with water indicating a successful
separation of the particles and plasma. Nevertheless,
the detection is dependent on the resolution of the
acquired images. Consequently, the minimum value,
corresponding to the maximum amount of particles,
obtained in each measurement varied. Further
techniques and models for image acquisition and data
treatment need to be improved for a better evaluation
of the effectiveness of the device.
4 CONCLUSIONS
The present study shows the potentiality of a novel
geometry for a microfluidic device for the separation
of blood cells and plasma. The separation of the
microparticles from the base fluid was mainly due to
the successive contractions effect, where the particles
tend to migrate into the center whereas the base fluid
tend to flow towards the walls of the microchannel.
From the two manufacture processes tested, the
micromilling process without bonding showed
promising results with grey values close to the ones
obtained with water. The depth of the channels and
the size of the particles did not show a major influence
on the separation process. On the other hand, the fluid
flow affected the results obtained, being 100 μL/min
the flow that presented better results. Further image
analysis studies and optimization of the geometry to
improve the separation process will be performed in
the near future.
ACKNOWLEDGEMENTS
Authors acknowledge FCT – Fundação para a Ciência
e a Tecnologia for partially supporting this
work through projects UIDB/00319/2020,
UIDB/04077/2020, UIDB/00690/2020, UIDB/
04436/2020 and PTDC/EME-SIS/30171/2017
NORTE-01-0145-FEDER-030171, and NORTE-01-
0145-FEDER-029394 funded by COMPETE2020,
NORTE 2020, PORTUGAL 2020, Lisb@2020 and
FEDER and for supporting the PhD Fellowship
(2020.08646.BD).
REFERENCES
Faustino, V.; Rodrigues, R.O.; Pinho, D.; Costa, E.; Santos-
Silva, A.; Miranda, V.; Amaral, J.S.; Lima, R. A (2019),
Microfluidic Deformability Assessment of Pathological
Red Blood Cells Flowing in a Hyperbolic Converging
Microchannel. Micromachines, 10, 645.
Yin, X., Thomas, T., Zhang, J. (2013) Multiple red blood
cell flows through microvascular bifurcations: Cell free
layer, cell trajectory, and hematocrit separation.
Microvasc. Res., 89:47–56.
Shamloo, A., Vatankhah, P., Bijarchi, M.A. (2016)
Numerical optimization and inverse study of a
microfluidic device for blood plasma separation. Eur. J.
Mech. B/Fluids, 57: 31–39.
Nam, J., Namgung, B., Lim, C.T., Bae, J.E., Leo, H.L.,
Cho, K.S., Kim, S. (2015) Microfluidic device for
sheathless particle focusing and separation using a
viscoelastic fluid. J. Chromatogr. A, 1406:244–250.
Lee, M.G., Shin, J.H., Choi, S., Park, J.K. (2014) Enhanced
blood plasma separation by modulation of inertial lift
force. Sensors Actuators, B Chem., 190:311–317.
Al-Fandi, M., Al-Rousan, M., Jaradat, M.A.K., Al-Ebbini,
L. (2011) New design for the separation of
microorganisms using microfluidic deterministic lateral
displacement. Robot. Comput. Integr. Manuf., 27:237–
244.
Bento, D., Fernandes, C.S. Miranda, J.M., Lima, R. (2019)
In vitro blood flow visualizations and cell-free layer
(CFL) measurements in a microchannel network. Exp.
Therm. Fluid Sci., 109:109847.
Catarino, S. O., R. O. Rodrigues, D. Pinho, J. M. Miranda,
G. Minas, R. Lima. (2019) Blood Cells Separation and
Sorting Techniques of Passive Microfluidic Devices:
From Fabrication to Applications. Micromachines, 10,
593.
Pinho D, Carvalho V, Gonçalves IM, Teixeira S, Lima R.
(2020) Visualization and Measurements of Blood Cells
Flowing in Microfluidic Systems and Blood Rheology:
A Personalized Medicine Perspective Journal of
Personalized Medicine 10, 4, 249.
Nam, J., Yoon, J., Kim, J., Jang, W.S., Lim, C.S. (2019)
Continuous erythrocyte removal and leukocyte
separation from whole blood based on viscoelastic cell
focusing and the margination phenomenon. J.
Chromatogr. A, 1595:230–239.
Geng, Z., Ju, Y., Wang, W., Li, Z. (2013) Continuous blood
separation utilizing spiral filtration microchannel with
gradually varied width and micro-pillar array. Sensors
Actuators, B Chem., 180:122–129.
Tsutsui, H., Ho, C.M. (2009) Cell separation by non-inertial
force fields in microfluidic systems. Mech. Res.
Commun., 36:92–103.
Ookawara, S., Oozeki, N., Ogawa, K., Löb, P., Hessel, V.
(2010) Process intensification of particle separation by
Separation Microfluidic Devices Fabricated by Different Milling Processes
189