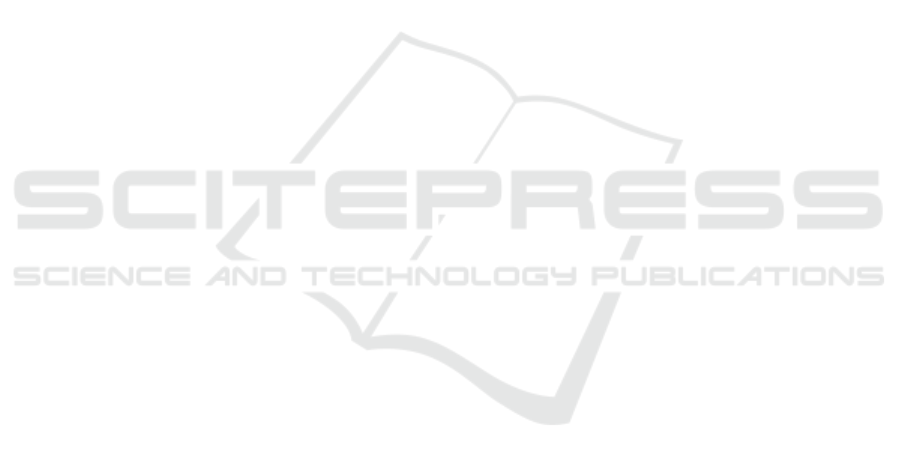
the accompanying increase in living standards
(Meckling, 2020). Over the past two centuries, the
world economy has been heavily dependent on the
overexploitation of natural resources and changes in
the life-sustaining biogeochemical cycles and
processes in the biosphere. The current boom in oil
exploitation and deforestation is a response to
pressure to meet growing demand for energy, food
and other commodities. These environmentally
unfriendly practices are the main reasons for
increasing emissions of anthropogenic sources of
global greenhouse gases (GHGs), the main drivers of
climate change. In 2016, energy and food systems
accounted for more than 90% of all global GHG
emissions (mostly as CO2) (Vladimirov, 2019). GHG
emissions are expected to increase by 50% by 2050,
mainly due to the expected 70% increase energy-
related CO2 emissions (Molchanova, 2019). If these
emissions continue to rise at their current rate, it will
throw the carbon (C) cycle out of dynamic
equilibrium, causing irreversible changes to the
climate system. Therefore, a concerted effort needs to
be made to reduce carbon emissions and increase
carbon sequestration through various socio-economic
and technological interventions. In response to the
ever-increasing global greenhouse effect, all
countries signed the landmark United Nations
Climate Agreement in Paris in December 2015 to
jointly tackle greenhouse gas emissions and combat
climate change. Under the 2015 Paris Agreement, all
countries agreed to keep warming below 2.0°C and
make efforts to keep global warming below 1.5°C by
achieving carbon neutrality by 2050 (Egorova, 2020).
The average global temperature in 2020 was 1.2°C
warmer than pre-industrial temperatures, and the
effects of this warming are being felt around the
world. Based on current climate data, there is an
urgent need to step up our efforts to reduce
atmospheric concentrations of greenhouse gases in
order to reverse global climate change.
To achieve carbon neutrality and sustainably
support human activities, it is essential to reduce
carbon emissions from fossil fuels and food while
promoting carbon sequestration in terrestrial and
marine ecosystems. Different strategic paths have
been mapped out in different countries to achieve
carbon neutrality, but due to the magnitude of the
flows involved, reducing carbon emissions to zero is
a challenging task (Meckling, 2020). According to the
International Energy Agency, if the world becomes
carbon neutral by 2050, the extraction and
development of new deposits of crude oil, natural gas
and coal should cease in 2021. In this regard,
investment in research and implementation of
renewable energy sources from non-carbon sources
(i.e. sunlight, tides, wind, water, waves, rain and
geothermal energy) and biomass (i.e. organic
materials from plants or animals) are the key to
bridging the gap between rhetoric and reality about
zero CO2 emissions.
Renewable resources can provide more than 3,000
times more than current global energy demand. The
global demand for renewable energy sources (in the
form of electricity, heat and biofuels) has grown
significantly over the past decade, with the share of
renewable energy sources in global electricity
production rising from 27% in 2019 to 29% in 2020.
Despite this progress in the use of renewable energy
sources, the pace of transition from traditional to
renewable energy sources is not high enough, and the
world is not on track to achieve carbon neutrality and
sustainable development by 2050 (Gakaev, 2020).
Therefore, additional efforts are needed to convert
energy. sector into a climate-neutral hub. This can be
achieved through the joint work of various
interdisciplinary research groups and the application
of integrated approaches developed as a result of the
latest scientific and technical achievements in the
field of civil engineering and ecology, biotechnology,
nanotechnology and other fields. In addition to the
development of renewable energy sources, there is
also a need to optimize the management of food
systems in order to improve production efficiency and
reduce carbon emissions. This can be achieved
through the development of new technologies for
more efficient fertilizer production and precision
farming, the integration of crop and livestock
systems, and the development of carbon neutral food
production systems. Given that the world is unlikely
to significantly reduce fossil fuel-based CO2
emissions in the short term, harnessing the power of
natural resources and processes to remove CO2 from
the atmosphere represents a viable path to carbon
neutrality. To mitigate climate change, various
potential strategies are being explored to increase
industrial carbon capture from the atmosphere and
carbon sequestration in terrestrial and marine
ecosystems.
These include bioenergy with carbon capture and
storage; increased weathering of rocks due to the
spread of crushed minerals, which are naturally able
to absorb CO2 on land or in the ocean; afforestation
and reforestation; carbon sequestration in the soil
with biochar, compost, direct application of biowaste
and conservation tillage, among others; ocean
fertilization through the use of iron and/or other
nutrients to stimulate the growth of photosynthetic
plankton; restoration of coastal wetlands; and direct
MMTGE 2022 - I International Conference "Methods, models, technologies for sustainable development: agroclimatic projects and carbon
neutrality", Kadyrov Chechen State University Chechen Republic, Grozny, st. Sher
28