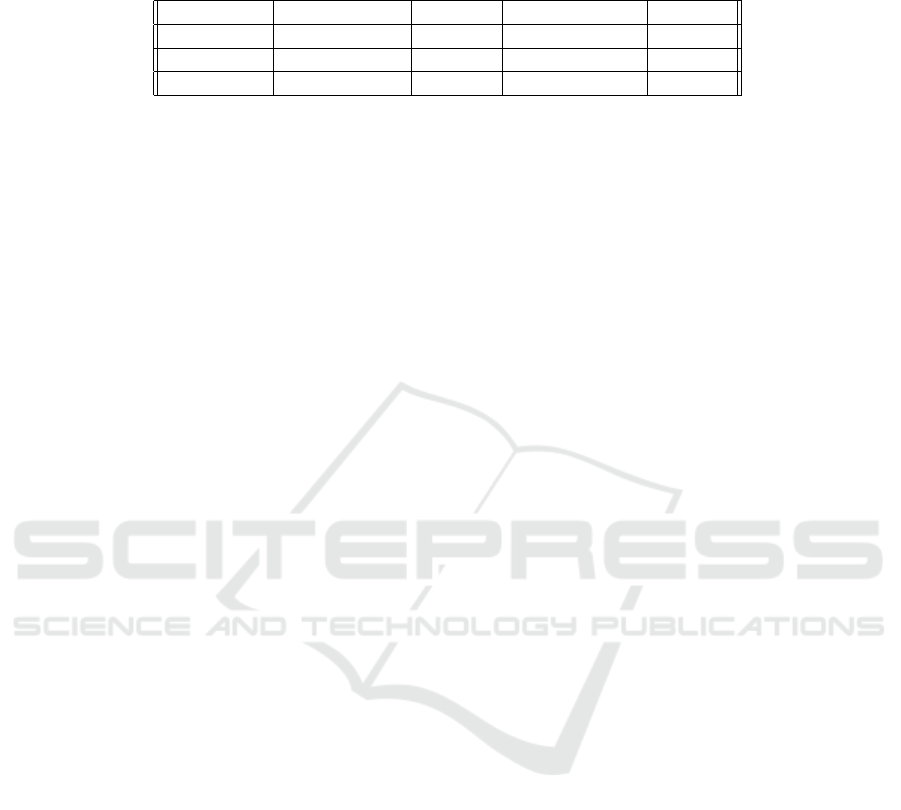
Table 2: Table showing how the number of tracks generated for the human prostate data set reduce as the strictness of the
continuity range increases, presented as the raw number of tracks and also as percentage of the tracks generated in R1. The
tracks in the cancer and healthy regions have been separated to show the difference in behaviour.
Cancer Tracks % of R1 Healthy Tracks % of R1
R1 10386 100 46832 100
R2, γ = 1.5 7309 70.37 35379 75.54
R2, γ = 1 2569 24.74 15717 33.56
linking between vessels and the first link in a track
identified in current particle linking approaches and
methods when applied to CEUS images. In synthetic
data, we have shown that PTNS improves the perfor-
mance of particle tracking. PTNS uses the data more
efficiently as a result of increasing the precision and
Jaccard index. In animal data, we have demonstrated
that PTNS can construct complex structures of in vivo
data, particularly in regions of high MB density.
Finally, we have applied PTNS to human prostate
data to investigate the structure and dynamics of the
vasculature for a patient with prostate cancer. Be-
cause PTNS is a two run process, the second run can
be used to probe different levels of motion continuity
by varying the control parameters, which can provide
the means to distinguish different blood flow dynam-
ics between cancer and healthy tissue. Using this ap-
proach, we observe that the cancer region exhibited a
more chaotic vascular structure, resulting in a larger
decrease in track number when the velocity continu-
ity was made more restrictive. This is consistent with
the current understanding of prostate cancer. More
broadly, this result shows that super resolution ultra-
sound imaging of prostate cancer can potentially be
developed into tools for diagnosis and focal therapy.
ACKNOWLEDGEMENTS
We would like to thank Lantheus Medical Imaging,
Inc. for providing the Luminity
®
contrast agent as part
of their Research Grants program. We would also like
to thank the Western General Hospital Edinburgh the-
atre team for their time and for allowing us to use their
facilities.
REFERENCES
Alizadeh, F., Hadi, M., Khorrami, M., Yazdani, M.,
Joozdani, R., Tadayyon, F., and Mellat, M. (2013).
Prostate cancer: Relationship between vascular diam-
eter, shape and density and gleason score in needle
biopsy specimens. Advanced Biomedical Research,
2(1).
Betzig, E., Patterson, G., Sougrat, R., Lindwasser, O.,
Olenych, S., Bonifacino, J., Davidson, M., Lippincott-
Schwartz, J., and Hess, H. (2006). Imaging intracellu-
lar fluorescent proteins at nanometer resolution. Sci-
ence, 313(5793):16–42–1645.
Brown, J., Christensen-Jeffries, K., Harput, S., Zhang,
G., Zhu, J., Dunsby, C., Tang, M., and Eckersley,
R. (2019). Investigation of microbubble detection
methods for super-resolution imaging of microvas-
culature. IEEE Transactions on Ultrasonics, Ferro-
electrics, and Frequency Control, 66(4):676–691.
Chenouard, N., Smal, I., de Chaumont, F., Ma
ˇ
ska, M.,
Sbalzarini, I., Gong, Y., Cardinale, J., Carthel, C.,
Coraluppi, S., Winter, M., Cohen, A., Godinez, W.,
Rohr, K., Kalaidzidis, Y., Liang, L., Duncan, J., Shen,
H., Xu, Y., Magnusson, K., Jald
´
en, J., Blau, H., Paul-
Gilloteaux, P., Roudot, P., Kervrann, C., Waharte, F.,
Tinevez, J., Shorte, S., Willemse, J., Celler, K., van
Wezel, G., Dan, H., Tsai, Y., de Sol
´
orzano, C., Olivo-
Marin, J., and Meijering, E. (2014). Objective com-
parison of particle tracking methods. Nature Methods,
11(3):281–289.
Couture, O., Hingot, V., Heiles, B., Muleki-Seya, P.,
and Tanter, M. (2018). Ultrasound localisation mi-
croscopy and super resolution: a state of the art. IEEE
Transactions on Ultrasonics, Ferroelectrics, and Fre-
quency Control, 65(8):1304–1320.
Errico, C., Pierre, J., Pezet, S., Desailly, Y., Lenkei, Z., Cou-
ture, O., and Tanter, M. (2015). Ultrafast ultrasound
localisation microscopy for deep super-resolution vas-
cular imaging. Nature, 527(7579):499–502.
Forster, J., Harriss-Philips, W., Douglass, M., and Bezak, E.
(2017). A review of the development of tumor vascu-
lature and its effects on the tumor microenvironment.
Hypoxia, 5:21–32.
Genovesio, A., Liedl, T., Emiliani, V., Parak, W., Coppey-
Moisan, M., and Olivo-Marin, J. (2006). Multiple par-
ticle tracking in 3-d+t microscopy: method and appli-
cation to the tracking of endocytosed quantum dots.
IEEE Transactions on Image Processing, 15(5):1062–
1070.
Godinez, W., Lampe, M., W
¨
orz, S., M
¨
uller, B., Eils, R.,
and Rohr, K. (2009). Deterministic and probabilistic
approaches for tracking virus particles in time-lapse
fluorescence microscopy image sequences. Medical
Image Analysis, 13(2):325–342.
Hess, S., Girirajan, T., and Mason, M. (2006). Ultra-
high resolution imaging by fluorescence photoactiva-
tion localisation microscopy. Biophysical Journal,
91(11):4258–4272.
Jochumsen, M., S
¨
orensen, J., Pederson, B., Nyengaard, J.,
Krag, S., Frøkiær, J., Borre, M., Bouchelouche, K.,
Particle Tracking with Neighbourhood Similarities: A New Method for Super Resolution Ultrasound Imaging
39