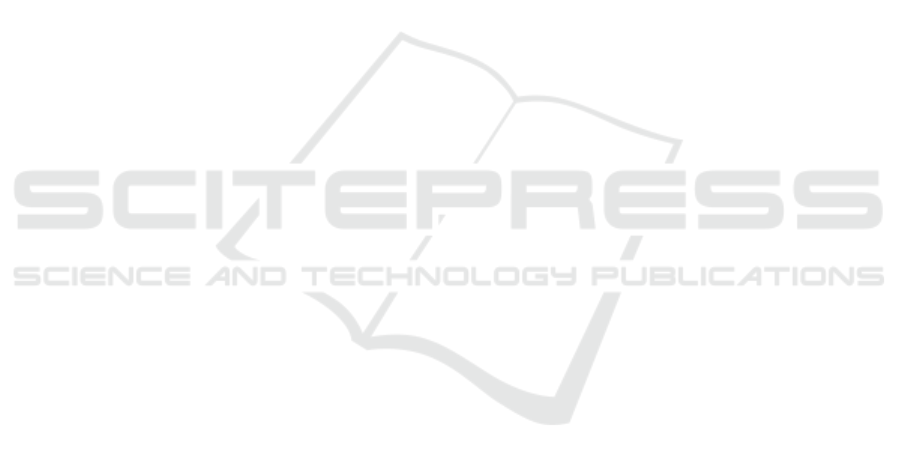
Integration of the Reflex Pharyngeal Swallow Into
Rhythmic Oral Activity in a Neurologically Intact Pig
Model. Journal of Neurophysiology, pages 1017–
1025.
Hamlet, S. L., Nelson, R. J., and Patterson, R. L. (1990).
Interpreting the Sounds of Swallowing: Fluid Flow
through the Cricopharyngeus. Annals of Otology, Rhi-
nology & Laryngology, 99:749–752.
Kurosu, A., Coyle, J. L., Dudik, J. M., and Sejdic, E. (2019).
Detection of Swallow Kinematic Events From Acous-
tic High-Resolution Cervical Auscultation Signals in
Patients With Stroke. Archives of Physical Medicine
and Rehabilitation, pages 501–508.
Kurt, T., G
¨
urg
¨
or, N., Sec¸il, Y., Yıldız, N., and Ertekin, C.
(2006). Electrophysiologic identification and evalu-
ation of stylohyoid and posterior digastricus muscle
complex. Journal of Electromyography and Kinesiol-
ogy, 16:58–65.
Lee, J., Steele, C. M., and Chau, T. (2008). Time and
time–frequency characterization of dual-axis swal-
lowing accelerometry signals. Physiological Mea-
surement, page 1105.
Li, X., Zhou, P., and Aruin, A. S. (2007). Teager–Kaiser
Energy Operation of Surface EMG Improves Muscle
Activity Onset Detection. Annals of Biomedical Engi-
neering, pages 1532–1538.
Lippert, D., Hoffman, M. R., Britt, C. J., Jones, C. A.,
Hernandez, J., Ciucci, M. R., and McCulloch, T. M.
(2016). Preliminary Evaluation of Functional Swal-
low After Total Laryngectomy Using High-Resolution
Manometry. Annals of Otology, Rhinology & Laryn-
gology, 125:541–549.
Maclean, J., Cotton, S., and Perry, A. (2009). Post-
Laryngectomy: It’s Hard to Swallow. Dysphagia,
pages 172–179.
McKeown, M. J., Torpey, D. C., and Gehm, W. C. (2002).
Non-invasive monitoring of functionally distinct mus-
cle activations during swallowing. Clinical Neuro-
physiology, pages 354–366.
Morini
`
ere, S., Boiron, M., Alison, D., Makris, P., and Beut-
ter, P. (2008). Origin of the Sound Components Dur-
ing Pharyngeal Swallowing in Normal Subjects. Dys-
phagia, pages 267–273.
Okada, T., Aoyagi, Y., Inamoto, Y., Saitoh, E., Kagaya, H.,
Shibata, S., Ota, K., and Ueda, K. (2013). Dynamic
change in hyoid muscle length associated with trajec-
tory of hyoid bone during swallowing: analysis using
320-row area detector computed tomography. Journal
of Applied Physiology, pages 1138–1145.
Park, D., Lee, H. H., Lee, S. T., Oh, Y., Lee, J. C.,
Nam, K. W., and Ryu, J. S. (2017). Normal contrac-
tile algorithm of swallowing related muscles revealed
by needle EMG and its comparison to videofluoro-
scopic swallowing study and high resolution manom-
etry studies: A preliminary study. Journal of Elec-
tromyography and Kinesiology, pages 81–89.
Pearson, W. G., Langmore, S. E., and Zumwalt, A. C.
(2011). Evaluating the Structural Properties of
Suprahyoid Muscles and their Potential for Moving
the Hyoid. Dysphagia, pages 345–351.
Selvan, S. E., Allexandre, D., Amato, U., and Yue, G. H.
(2018). Unsupervised Stochastic Strategies for Ro-
bust Detection of Muscle Activation Onsets in Surface
Electromyogram. IEEE Transactions on Neural Sys-
tems and Rehabilitation Engineering, 26:1279–1291.
Shaw, S. M. and Martino, R. (2013). The Normal Swallow:
Muscular and Neurophysiological Control. Otolaryn-
gologic Clinics of North America, pages 937–956.
Steele, C. M. (2015). The Blind Scientists and the Elephant
of Swallowing: A Review of Instrumental Perspec-
tives on Swallowing Physiology. Journal of Texture
Studies, pages 122–137.
Takahashi, K., Groher, M. E., and Michi, K.-i. (1994).
Methodology for detecting swallowing sounds. Dys-
phagia, pages 54–62.
Tenan, M. S., Tweedell, A. J., and Haynes, C. A. (2017).
Analysis of statistical and standard algorithms for de-
tecting muscle onset with surface electromyography.
PLOS ONE, page e0177312.
Thexton, A. J., Crompton, A. W., and German, R. Z. (2012).
EMG activity in hyoid muscles during pig suckling.
Journal of Applied Physiology, pages 1512–1519.
Xu, Q., Quan, Y., Yang, L., and He, J. (2013). An Adaptive
Algorithm for the Determination of the Onset and Off-
set of Muscle Contraction by EMG Signal Processing.
IEEE Transactions on Neural Systems and Rehabilita-
tion Engineering, pages 65–73.
BIOSIGNALS 2023 - 16th International Conference on Bio-inspired Systems and Signal Processing
54