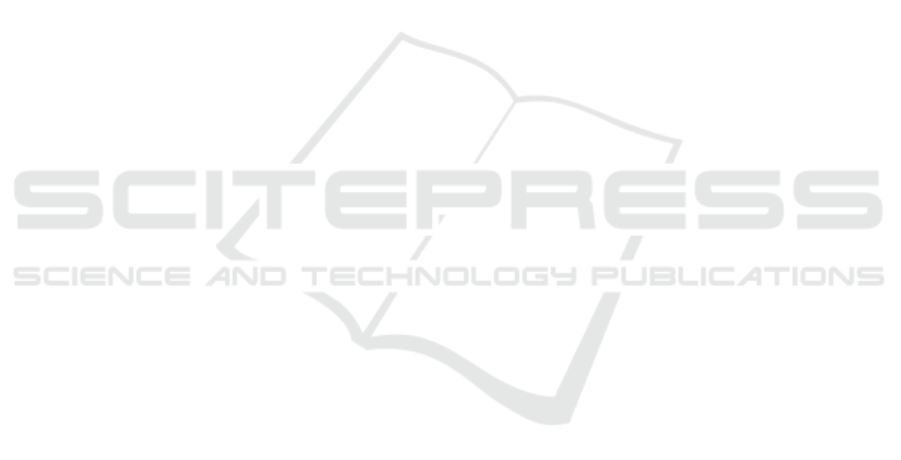
in this paper can reconstruct images even when the
signal-to-noise ratio is low due to scattering, using the
ability of GI. By using this method, target images can
be obtained without scattering effects.
2 DIFFERENT OF
CONVENTIONAL OCT AND
GI-OCT
Unlike conventional OCT, the OCT probe light from
GI-OCT does not focus on a point but illuminates the
light on an area, as in full field-OCT(FF-OCT). This
method can take two-dimensional images with a sin-
gle probe. FF-OCT enables imaging of target dis-
tribution because the camera directly takes a cross-
sectional two-dimensional image perpendicular to the
optical axis. However, FF-OCT cannot obtain tar-
get distribution without the effect of scattering. FF-
OCT uses a megapixel camera to achieve cellular-
level imaging. Each pixel in an FF-OCT image is a
point measurement of the target, the same as conven-
tional OCT(Vabre et al., 2002).
For ease of understanding, we show the target
(scattered sample) in the scattering media in two parts
on the OCT measurement. The former is the scat-
ter layer before the light hits the target, and the latter
is the target layer. When measuring the target layer
in the scatter media with conventional OCT, a three-
dimensional image can be constructed by changing
the reference light path (A scan) and orthogonal mo-
tion of the probe (B scan) as shown in Figure 1(a).
However, the reflected signal does always have scat-
tering from the scatter layer, so the target layer dis-
tribution had modulated by scattering. For example,
when measuring the optical properties (transmittance
and absorbance) of a target layer with OCT, the dis-
tribution of the front scatter layer may change the di-
rection of the light or delay the received signal of the
target layer due to scattering. As a result, the modu-
lated image of the optical properties of the target layer
is detected.
Figure 1(b) illustrates the concept of GI-OCT, in
which GI-OCT probe light passes through an ex-
pander and spatial light modulator (SLM), and the
generated light patterns illuminate the target layer be-
hind the scatter layer. Reflected light from the tar-
get and scatter layers are focused on a point detec-
tor. The A-scan signal of GI-OCT is a series of light
intensities separated in the depth direction for each
light illuminated pattern. Every A-scan signal can be
separated as the sum of the illuminate pattern inten-
sities for each layer distribution with the axial resolu-
tion of OCT. After repeated measurements with dif-
ferent light patterns, the correlation between those il-
luminated patterns and the sum of interference inten-
sities of each separated layer is calculated by using
the GI method to obtain each layer distribution. Each
separated layer is reconstructed in a multilayer sam-
ple(3D).
Compared to conventional OCT measurements,
GI-OCT acquires a two-dimensional image in the
plane perpendicular to the optical axis with a sin-
gle detector. Light misalignment to other directions
and time delay in scattering media can be simultane-
ously summed and detected as OCT interference sig-
nals. The misalignment and delay are constructed as a
scatter layer distribution. The target layer distribution
and the former scattering layer distribution can be re-
constructed using the same procedure. By the scatter
layer distribution, the optical properties of the target
layer can be corrected, and the target distribution is
obtained without the scatter layer effects.
3 EXPERIMENT
Figure 3(a) shows the GI-OCT experiment setup.
The GI-OCT uses the superluminescent diodes (SLD)
light source with a central wavelength of 860 nm,
Gaussian distribution, and an axial resolution of
10.1µm. The reference path consists of a steady ro-
tating motor and a fixed mirror to complete the axial
scanning (A-scan)(Shiina et al., 2003). The measure-
ment path of GI-OCT has the optical probe, which
is composed of a collimator and two lenses to make
the beam diameter expand. The DMD chip (DLP2000
DMD) is placed in the measurement area. The sample
is set between the DMD chip and the optical probe.
There is a matrix corresponding to 640 × 360
and a total size of 4.84 × 2.72mm micromirrors ar-
ranged inside the DMD chip. Each mirror size is
7.56 × 7.56 µm, and the deflection angle is ±12 de-
grees on the diagonal axis, divided into ”on” and ”off”
states. Then, the DMD chip in our experiments is ori-
ented towards the vertical optical axis 12 degree to
obtain the reflected light from the DMD. DMD chips
are rotated 45 degrees counterclockwise in the verti-
cal plane against the optical axis to make the diagonal
axis vertical too. Moreover, the DMD is tilted 12 de-
gree against the optical axis when the micromirrors
state is ”on”. Because of these tilts, this DMD ori-
entation causes a series of interference signals in the
time domain, as shown in Fig 3(b). In GI-OCT ex-
periment, the DMD chip displays 10 × 10 speckles
patterns in the area, which is 2 × 2mm in size.
We prepare a sample with the target in the scatter-
PHOTOPTICS 2023 - 11th International Conference on Photonics, Optics and Laser Technology
68