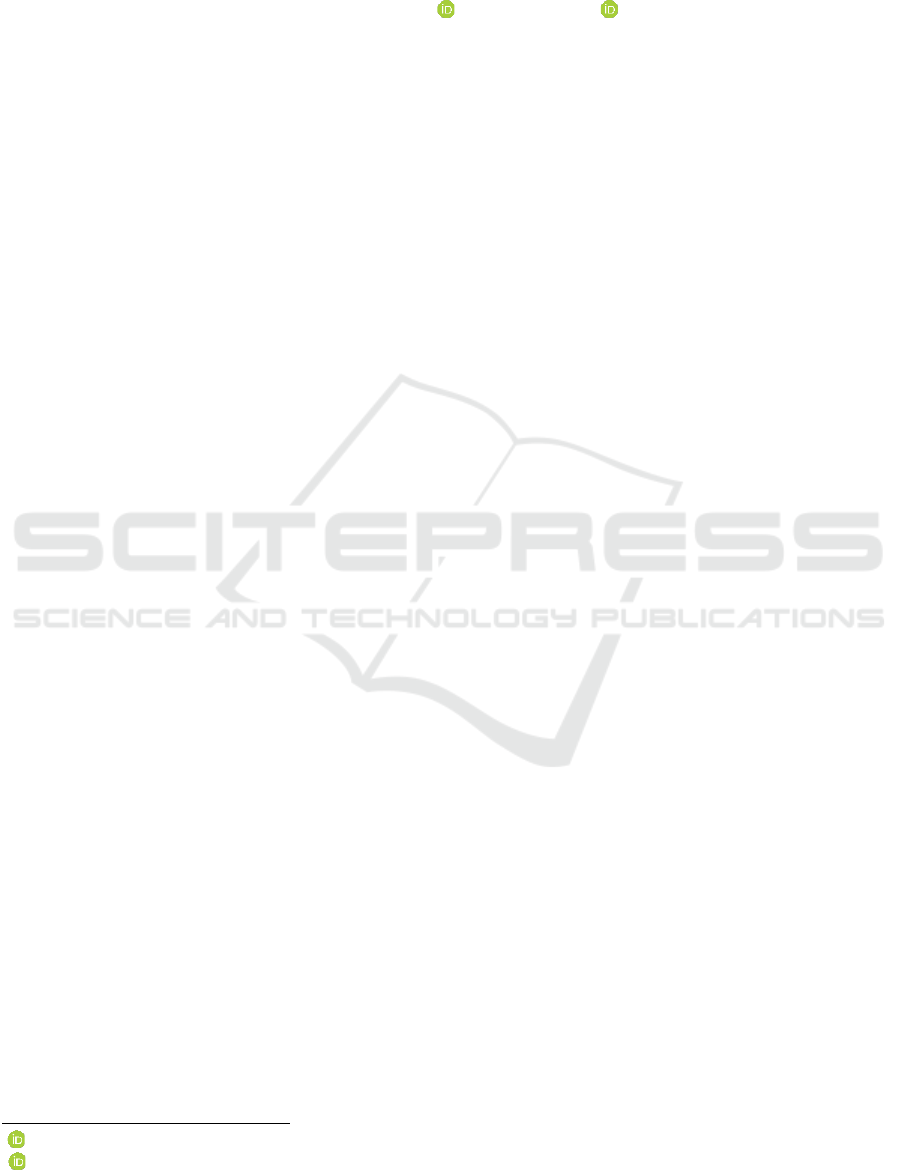
Effective Multilevel Inverter with 129 Level Output
A. Annai Theresa
1 a
and S. Malathi
2 b
1
Anna University, SRM Valliammai Engineering College, Chennai, India
2
Department of Electrical and Electronics Engineering, SRM Valliammai Engineering College, Chennai, India
Keywords: Multilevel Inverter (MLI), 129 Level Asymmetrical Cascaded Multilevel DC Source Inverter (ASCMLDCSI),
Total Harmonic Distortion (THD), MATLAB/ SIMULINK.
Abstract: The multilevel inverter (MLI) is a power conversion scheme that provides the desired alternating current level
by utilizing numerous DC sources. Applications requiring medium to high power can use it. For getting a
pure sinewave in an effective manner, this paper showcases a 129-level asymmetrical cascaded multilevel DC
source inverter (ASCMLDCSI) with lower switching components and lower total harmonic distortion (THD).
Multiple DC sources with voltage ratios of 1:1:2:4:8:12:16 are used in the suggested inverter. To regulate the
switching components of the architecture, the suggested inverter employs a voltage reference approach. The
suggested topology has several advantages, including smaller switching components, fewer losses, and lower
THD. To simulate and analyse the performance of the suggested topology, MATLAB/SIMULINK software
is employed.
1 INTRODUCTION
Because of the benefits of high quality and clear
power output wave forms, low interference with the
electronic signals, low losses in switching, and
capability of withstanding high-voltage,
MULTILEVEL power conversion has grown in
popularity in recent years. A staggering amount of
semiconductor devices required is the fundamental
downside of this technique. Because lower-voltage
devices can be utilized, this does not result in a
considerable cost rise. However, we need a more
intricate mechanical architecture and more gate drive
electronics. Although the diode clamped multilevel
inverter has garnered a lot of attention in the
literature, the cascading or series-connected H-bridge
inverter topologies have received a lot of interest as
well [J. Rodríguez, 2002].
The primary benefit of this design is its simplicity,
as well as the possibility to cascade fewer or more H-
bridge cells to reduce or improve voltage and power
levels, respectively. The primary drawback of this
design is that a single H-bridge cell needs its own
independent DC supply. Separated sources are often
powered via a transformer/rectifier arrangement, but
a
https://orcid.org/0009-0004-5127-5140
b
https://orcid.org/0000-0001-7703-0281
they can also be powered by batteries, capacitors, or
photovoltaic arrays [K.T. Maheswari, 2021].
Robicon Group recently patented this topology in
1996, and it is now one of the company's regular drive
solutions. Utilizing distinct DC voltages on each
series H-bridge to expand the number of voltage
levels and enhance power quality is one of the most
recent advancements in cascaded H-bridge inverters.
Only in 1998 and 1999 was this invention granted
patent protection [Keith Corzine, 2002]. Then the
researchers started to develop a special kind of
inverters like cascading the H- bridge cells with the
ability to switch between more number of levels. The
cascading technology mushroomed in the research
field and the H- bridge inverters developed with a
large number of levels, less THD, minimum number
of drivers and switching components, simple
operational process, compact circuits, optimum
losses and improved efficiency. Not only the
cascading technology, but also some other techniques
were implemented in the process of the development
of multilevel inverter technology [P. Omer, 2020].
This paper is an effort to improve the quality of the
output waveform by increasing the output to 129
Theresa, A. and Malathi, S.
Effective Multilevel Inverter with 129 Level Output.
DOI: 10.5220/0012509100003808
Paper published under CC license (CC BY-NC-ND 4.0)
In Proceedings of the 1st International Conference on Intelligent and Sustainable Power and Energy Systems (ISPES 2023), pages 93-99
ISBN: 978-989-758-689-7
Proceedings Copyright © 2024 by SCITEPRESS – Science and Technology Publications, Lda.
93