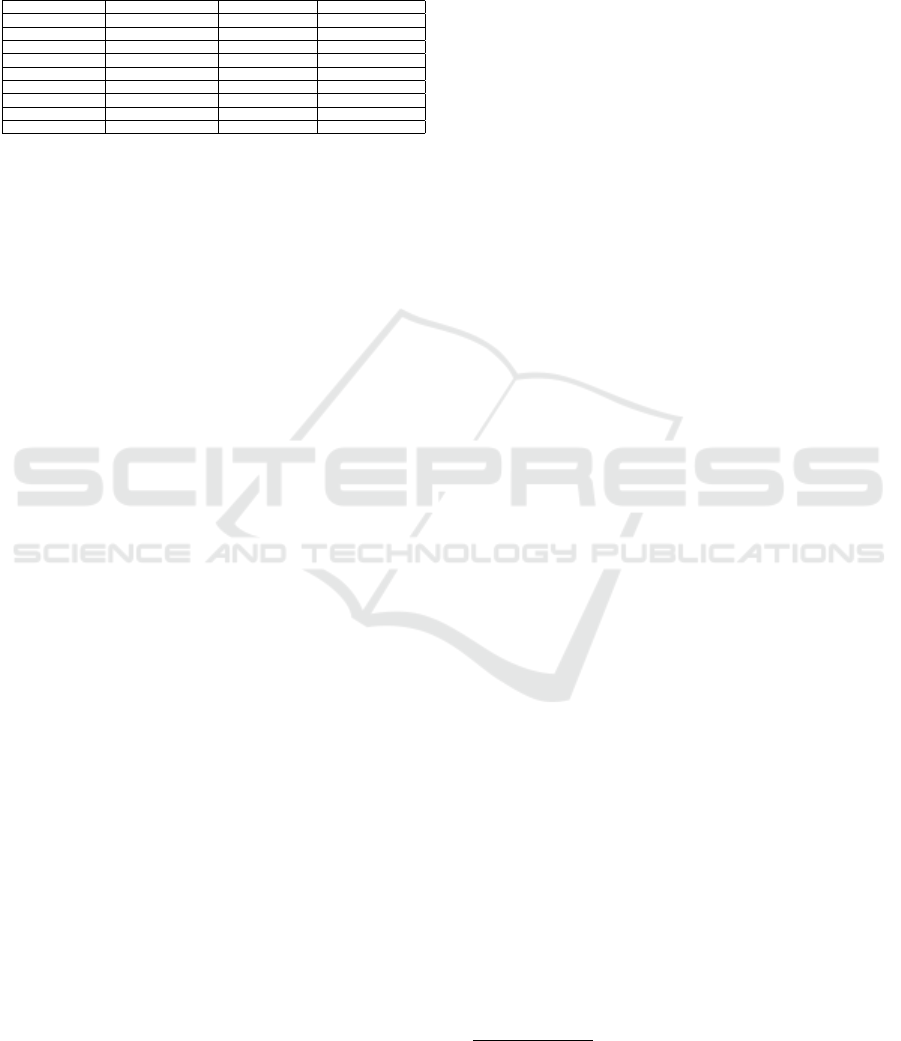
mainly from scientific articles and books related to the
considered domain. The Table 1 shows part of these
terms that make up the Domain Lexicon.
Table 1: Part of the terms that make up the Domain Lexi-
con.
Axial external force Factor of safety Member stiffness Stress
Bolt Fasteners Nut Tapped thread joint
Bolt tension Fine pitch thread Plain washer Thread
Bolted joint Geometry Regular nut Threaded connection
Clamped member Hexagonal nut Results Tool
Coarse pitch thread ISO thread SAE specifications UNC thread
Connection system Joint Screw UNF thread
Design Loads Simplified method UNS thread
Disassembly Material Size Variables
Element Mechanical properties Software Washers
The next step in this workflow consists of creating
the Reference Lexicon (LR), through the intersection
between the LA and the LD (terms common to both
lexicons). In addition, unique terms can be added to
each of the lexicons, which are considered important
by the Domain Expert (De Nicola et al., 2009). To
help with the task of identifying terms common to LA
and LD, another script was created in Python, with
the options to compare the two lexicons, as well as to
allow manual entry of terms. With the intersection of
the LA and LD terms, as well as adding some terms
manually, the LR was completed after creating seven
versions in .txt format and with a total of 58 terms.
The creation and intersection of lexicons aims to
identify the most important concepts of the consid-
ered domain, which will serve as a basis for building
the ontology, mainly in the form of classes and prop-
erties. Therefore, it is important that these terms are
formally defined, associating definitions from several
sources for each (De Nicola et al., 2009) term. Thus,
the next stage of this workflow dealt with the elabora-
tion of a Reference Glossary (GR), where a definition
was associated with each term. Table 2 shows a frac-
tion set of the GR. This workflow ends with the vali-
dation of the GR by verifying the scope of the defined
terms. Thus, there was a need to perform some iter-
ations, returning to LA and LD to find and add new
terms, as well as manually adding important terms to
LR, present in only one of the predecessor lexicons.
3.3 The Project Workflow
According to (De Nicola et al., 2009), this workflow
starts with the modeling of concepts, in which they
are organized into three primary categories and some
complementary categories. The primary categories
are: business actor (business actor), business object
(business object) and business process (business pro-
cess). In the business actor category, elements capa-
ble of activating, executing or monitoring a process
are identified. Entities in which a process operates are
allocated in a business object. As a business process,
activities or operations that aim to achieve a defined
objective (De Nicola et al., 2009) are classified. For
this work, the concepts present in the Table 3 were
listed for the primary categories, mainly coming from
the GR. As a business actor, a user of a possible appli-
cation that uses the knowledge modeled in the ontol-
ogy was identified. In a business object, entities were
listed that an application would use to request infor-
mation from the ontology or perform calculations. Fi-
nally, in the business process category, activities car-
ried out by the possible application were separated. In
addition to the primary categories, there are comple-
mentary categories, necessary for a rich ontological
representation of the considered domain.
The next step in this workflow deals with mod-
eling concept hierarchies, where formal relationships
begin to be adopted and a hierarchical approach must
be chosen. According to (De Nicola et al., 2009),
there are three usual approaches: from top to bottom,
starting from more general terms towards more spe-
cific ones; from bottom to top, starting with specifics
towards general; and center out, an approach that
combines the previous ones. The last (combined) ap-
proach is considered to be the most efficient because
it starts with the most relevant and informative con-
cepts located in the central area of the domain, creat-
ing generalizations and specifications from these (De
Nicola et al., 2009). With that, a semantic network of
concepts begins to be built, represented by a class dia-
gram to which object properties are also added, which
relate individuals belonging to a class to individuals
of another class (Antoniou and Harmelen, 2009).
Using the combined approach, the hierarchy of
concepts for the present work was initiated by a class
Joint and the respective subclasses TappedThread-
Joint and BoltedJoint, representing the categories of
joints with threaded hole and bolted joints. In ad-
dition, a joint must be composed of elements and
bolts, as well as nuts and washers, making it neces-
sary to use the object properties hasElement, hasS-
crewFastener and hasWasher, relating the class Joint
to the respective classes of these components. Sub-
classes of a superclass can also have object proper-
ties, so TappedThreadJoint has the properties has-
THE_TTJ
3
and hasSHE_TTJ
4
, whereas BoltedJoint
has hasSHE_BJ
5
, so that it is possible to distinguish
which elements each type of joint can contain (with
simple or threaded hole). A property like hasWasher
does not appear in subclasses of Joint as both can have
the same washers. On the other hand, hasNut is a
3
hasThreadedHoleElement_TappedThreadJoint.
4
hasSimpleHoleElement_TappedThreadJoint.
5
hasSimpleHoleElement_BoltedJoint.
ICEIS 2024 - 26th International Conference on Enterprise Information Systems
58