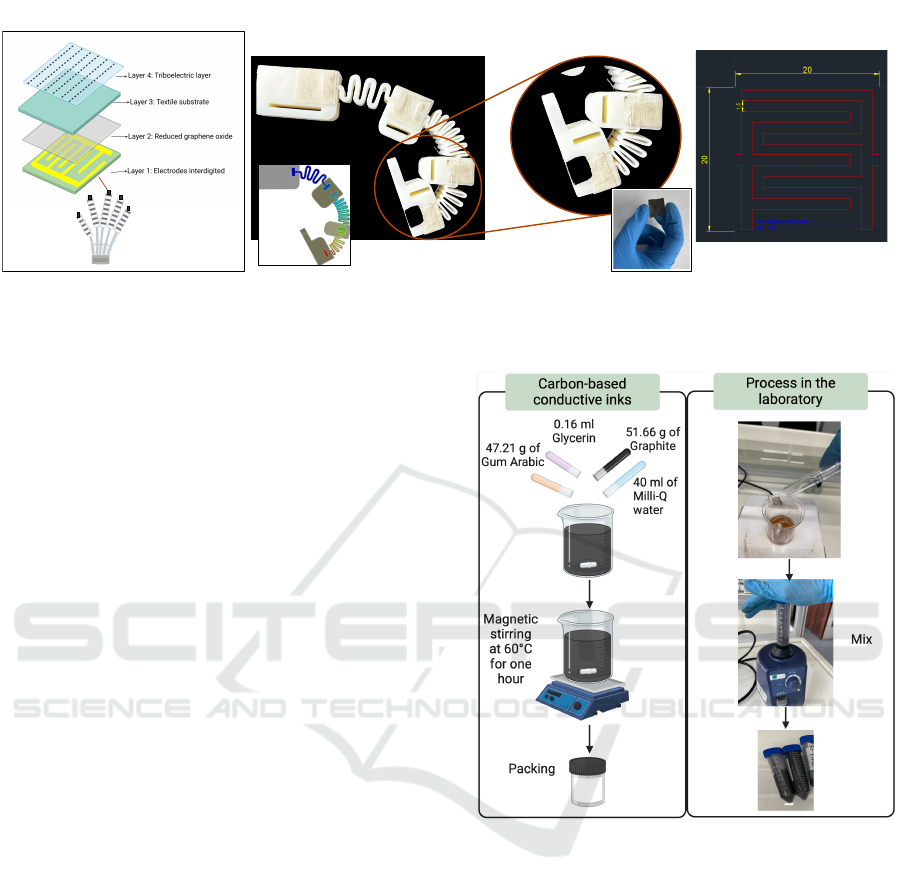
Soft exoskeleton
(compliant joints)
Bio-nanosensor
Caracterización
Sustrato de nanocelulosa
Fabricación de (Nanoparticulas de cobre)
Se sintetizan nanopartículas de cobre de 30
nm mediante el uso de ácido ascórbico.
Estas nanopartículas se utilizan para crear la
siguiente capa, que se aplicará sobre el
sustrato y entrará en contacto con la capa
de óxido de grafeno reducido. De esta
manera, se logra la construcción de un
sensor piezorresistivo que emula los
mecanorreceptores de adaptación lenta.
Fabricación de Nanomateriales (óxido de grafeno reducido)
• El objetivo es la fabricación de un sensor diseñado para imitar mecanorreceptores en
aplicaciones de electromiografía. Este sensor consta de múltiples capas y utiliza
nanomateriales, y está destinado a aplicarse en la piel para detectar dichas señales.
• Los nanomateriales son desarrollados utilizando métodos verdes o de bio-fabricación
con el objetivo de disminuir la toxicidad y aumentar la biocompatibilidad para
aplicaciones en medicina, en las Figuras A, B, C se realiza la primera fase de óxido de
grafeno y en la figura C y E se realiza la fabricación de óxido de grafeno reducido.
Fabricación de Sensores Basados en
Nanomateriales para Aplicaciones en
Electromiografía y Detección de
Esfuerzo
La creciente demanda de tecnologías avanzadas en el campo de la medicina y la
ingeniería ha impulsado la búsqueda de sensores altamente sensibles y versátiles. En
este contexto, la incorporación de nanomateriales en la fabricación de sensores ha
emergido como una solución prometedora. Presentamos una investigación centrada en
el desarrollo de sensores basados en nanomateriales diseñados específicamente para
medir señales electromiográficas (EMG) y esfuerzo para su aplicación en la creciente
área de la robótica blanda (soft robotics). Estos sensores ofrecen una combinación única
de sensibilidad, flexibilidad y adaptabilidad, lo que los convierte en herramientas ideales
para la detección precisa de señales biológicas y su integración en sistemas de control en
la robótica blanda.
!
Facultad de Ingeniería, Pontificia Universidad Javeriana, Bogotá, Colombia
"
Departamento de Física, Pontificia Universidad Javeriana Bogotá, Colombia.
Fredy A. Cuellar
1
, Julián D. Colorado
1
,
Catalina Alvarado-Rojas
1
, Juan Carlos Salcedo Reyes
2
, Hernán
Rodríguez Hernández
2
Introducción
Métodos
Resultados
Conclusiones
Rector
Vicerrector Académico
Vicerrector del Medio Universitario
Secretario General
FACULTAD DE M EDIC INA
Decana Académica
Decano del Medio Universitario
Directora de Carrera
Director de Posgrados
Secretaria de Facultad
Director General
Directores de Departamento
Directores de Instituto
Editor
Comité Editorial
Corrección de Estilo
Diseño, preprensa e impresión
La Revista Universitas Médica -
San Ignacio. Todo el material remitido es revisado por el Comité Editorial
sus autores.
Commons Reconocimiento 4.0 Internacional.
Rector
Vicerrector Académico
Vicerrector del Medio Universitario
Secretario General
FACU LTAD D E MEDI CI NA
Decana Académica
Decano del Medio Universitario
Directora de Carrera
Director de Posgrados
Secretaria de Facultad
Director General
Directores de Departamento
Directores de Instituto
Editor
Comité Editorial
Corrección de Estilo
Diseño, preprensa e impresión
La Revista Universitas Médica -
San Ignacio. Todo el material remitido es revisado por el Comité Editorial
sus autores.
Commons Reconocimiento 4.0 Internacional.
Somos una sede que pertenece al Hospital
Universitario San Ignacio, vinculado con el Instituto
de Envejecimiento de la Facultad de Medicina de la
Pontificia Universidad Javeriana y otras unidades
académicas como el Departamento de Psiquiatría y
Salud Mental. Ofrecemos atención integral,
humana, segura y eficiente a personas con
problemas cognoscitivos del comportamiento y
deterioro funcional.
Evaluamos, entrenamos,
asesoramos a familiares y cuidadores para el
manejo del paciente en casa, así como para su
permanencia activa en la sociedad.
Robotic Exoskeleton for smart
rehabilitation
cognoscitiva.
Musicoterapia.
SEGUIMIENTO
CUIDADOS A LARGO PLAZO
Con la apertura de la nueva sede, tendremos
una Unidad con cobertura para pacientes que
requieran una larga estancia, ofreciendo
condiciones de vivienda dignas de alta calidad.
Tendremos servicios dirigidos al cuidado de
adultos y personas mayores, principalmente
con trastornos cognitivos, así como
alteraciones del comportamiento y la conducta,
con condiciones que les impidan continuar
viviendo en su hogar, para la asistencia y
seguimiento de sus comorbilidades.
Se indica la fabricación un sensor multifuncional utilizando nanomateriales para replicar
mecanorreceptores. Los nanomateriales se obtienen mediante métodos sostenibles y
biocompatibles, lo que los hace adecuados para aplicaciones médicas y de robótica
blanda. Ofrecen sensibilidad, flexibilidad y adaptabilidad, lo que los hace ideales para
detectar señales biológicas y controlar dispositivos de robótica blanda.
Sensores Señal EMG
Aplicación Soft para
rehabilitación
Microscopio
de Fuerza Atómica
Interdigitated electrode layout
Flexible fabric
Figure 1: Bio-nanosensor design layers and integration with a hand-based soft robotic exoskeleton to support tactile pressure
sensing in grasping-driven rehabilitation.
icity and maximize its bio-compatibility with the hu-
man skin. The sensor has multiple layers to mimic cu-
taneous mechanoreceptors with piezoelectric and tri-
boelectric layers. Electrical characterization is pre-
sented, demonstrating the potential of these flexi-
ble sensing devices synthesized in a small footprint,
while providing accurate sensitivity and precision.
2 METHODS
Figure 1 depicts the multilayered architecture of the
sensor composed by 4 stacked layers. A flexible
fabric was used to allow proper integration with the
exoskeleton prototype developed in previous work
reported in (Bonilla et al., 2023). As shown, the
first two layers constitute the main piezoelectric sen-
sor. The interdigitated electrode structure, fabricated
through screen printing with carbon-based inks, im-
parts conductivity to the sensor.
A flexible textile substrate is employed, highlight-
ing the applicability of sustainable and flexible mate-
rials for wearable technology. This electrode is paired
with a layer of reduced graphene oxide, synthesized
using an environmentally friendly method, to improve
the sensor’s performance. The subsequent layer is a
triboelectric layer, integrated to enhance the sensor
with surface texturing capabilities, which are crucial
for exoskeletons designed for hand rehabilitation.
2.1 Piezoelectric Layer Fabrication
The fabrication process is described as follows:
• Substrate preparation: A flexible and durable tex-
tile substrate is selected for its suitability in wear-
able technologies.
Figure 2: Conductive ink manufacturing process.
• Electrode patterning: Interdigitated electrodes are
directly printed onto the textile substrate using
conductive carbon-based inks through the screen-
printing technique. This method allows precise
control over the electrode patterns and thick-
nesses. Figure 2 details the fabrication process of
these conductive inks, highlighting the formula-
tion and application steps involved.
• Graphene-oxide application: Reduced graphene
oxide, produced via an environmentally friendly
reduction process, is subsequently applied to the
interdigitated electrodes. This coating is crucial
for enhancing the piezoelectric properties of the
sensor. Figures 3 and 4, provide a detailed expla-
nation of the synthesis process for this nanomate-
rial.
Nanosensors for Soft Robotics Exoskeletons
641