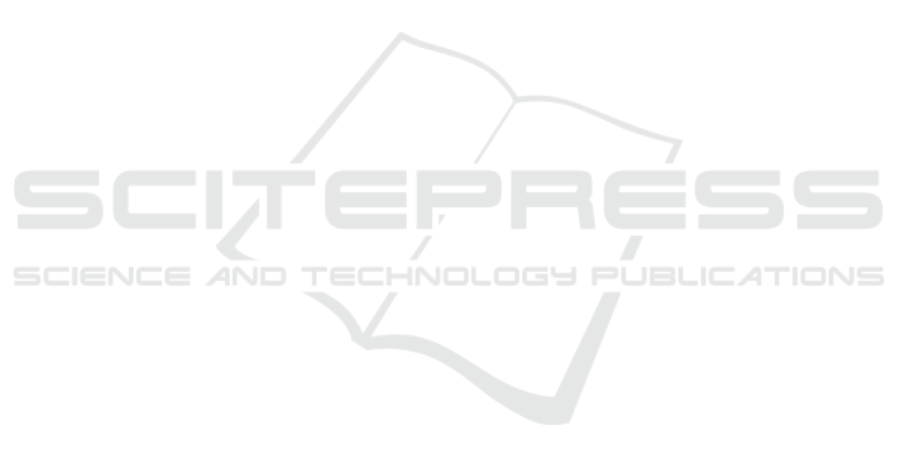
REFERENCES
Boorman, D. J., Mumaw, R. J., Pritchett, A., and Jackson,
A. (2004). A new autoflight/fms interface: Guiding
design principles. In Proceedings of the international
conference on human-computer interaction in aero-
nautics, pages 303–321.
Brooke, J. et al. (1996). Sus-a quick and dirty usability
scale. Usability evaluation in industry, 189(194):4–7.
Bureau d’enqu
ˆ
etes et d’Analyse, . . (1992). Accident sur-
venu le 20 janvier 1992 pr
`
es du mont sainte-odile (bas
rhin)
`
a l’airbus a 320 immatricul
´
e f-gged exploit
´
e par
la compagnie air inter. Technical report, BEA, FR.
Bureau of Air Safety Investigation, . . (1998). Advanced
technology aircraft safety survey report. Technical re-
port, BASI, AU.
Curry, R. E. (1985). The introduction of new cockpit tech-
nology: A human factors study. NASA TM 86659.
Denzin, N. K. (2009). The elephant in the living room: Or
extending the conversation about the politics of evi-
dence. Qualitative research, 9(2):139–160.
Eldredge, D., Mangold, S., and Dodd, R. S. (1992). A re-
view and discussion of flight management system in-
cidents reported to the aviation safety reporting sys-
tem. Technical Report 5855, U.S. Department of
Transportation, Bureau of Transportation Statistics.
Endsley, M. R. (1988). Situation awareness global assess-
ment technique (sagat). In Proceedings of the IEEE
1988 national aerospace and electronics conference,
pages 789–795. IEEE.
Feary, M., Alkin, M., Polson, P., McCrobie, D., Sherry, L.,
and Palmer, E. (1999). Aiding vertical guidance un-
derstanding. Air & Space Europe, 1(1):38–41.
Flanagan, J. C. (1954). The critical incident technique. Psy-
chological bulletin, 51(4):327.
Gugerty, L. J. (2017). Situation awareness during driving:
Explicit and implicit knowledge in dynamic spatial
memory. In Situational awareness, pages 379–404.
Routledge.
Hart, S. G. and Staveland, L. E. (1988a). Development
of nasa-tlx (task load index): Results of empirical
and theoretical research. In Hancock, P. A. and
Meshkati, N., editors, Human Mental Workload, vol-
ume 52 of Advances in Psychology, pages 139–183.
North-Holland.
Hart, S. G. and Staveland, L. E. (1988b). Development of
nasa-tlx (task load index): Results of empirical and
theoretical research. In Advances in psychology, vol-
ume 52, pages 139–183. Elsevier.
Hutchins, E. L. (1996). The Integrated Mode Management
Interface. NASA, USA.
Johnson, E. N. and Pritchett, A. R. (1995). Experimental
study of vertical flight path mode awareness. IFAC
Proceedings Volumes, 28(15):153–158.
Johnson, R. B. and Onwuegbuzie, A. J. (2004). Mixed
methods research: A research paradigm whose time
has come. Educational researcher, 33(7):14–26.
Jones, D. G. and Endsley, M. R. (1996). Sources of situa-
tion awareness errors in aviation. Aviation, space, and
environmental medicine, 67(6):507–512.
Kharoufah, H., Murray, J., Baxter, G., and Wild, G. (2018).
A review of human factors causations in commercial
air transport accidents and incidents: From to 2000–
2016. Progress in Aerospace Sciences, 99:1–13.
LeCompte, M. D. and Goetz, J. P. (1982). Problems of reli-
ability and validity in ethnographic research. Review
of educational research, 52(1):31–60.
Li, W.-C., White, J., Braithwaite, G., Greaves, M., and
Lin, J.-H. (2016). The evaluation of pilot’s situational
awareness during mode changes on flight mode an-
nunciators. In Harris, D., editor, Engineering Psy-
chology and Cognitive Ergonomics, pages 409–418,
Cham. Springer International Publishing.
Likert, R. (1932). A technique for the measurement of atti-
tudes. Archives of Psychology, 22(140):1–55.
Mackay, W. and McGrenere, J. (2024). Comparative struc-
tured observation. ACM Transactions on Computer-
Human Interaction (TOCHI), pages 1–25. to appear.
Mackay, W. E. and Beaudouin-Lafon, M. (2023). Participa-
tory design and prototyping. In Handbook of Human
Computer Interaction, pages 1–33. Springer.
Mackay, W. E. and Fayard, A.-L. (1997). Hci, natural sci-
ence and design: A framework for triangulation across
disciplines. In Proceedings of the 2nd Conference on
Designing Interactive Systems: Processes, Practices,
Methods, and Techniques, DIS ’97, page 223–234,
New York, NY, USA. Association for Computing Ma-
chinery.
Matthews, M. D. and Beal, S. A. (2002). Assessing situ-
ation awareness in field training exercises. US Army
Research Institute for the Behavioral and Social Sci-
ences, 31.
Mumaw, R., Boorman, D. J., and Prada, R. (2006). Ex-
perimental evaluation of a new autoflight interface.
In Proceedings HCI-Aero 2006, International Confer-
ence on Human Computer Interaction, Seattle, WA.
Mumaw, R. J. (2020). Addressing mode confusion using an
interpreter display. NASA Contractor Report.
Mumaw, R. J. (2021). Plan b for eliminating mode confu-
sion: An interpreter display. International Journal of
Human–Computer Interaction, 37(7):693–702.
Munir, A., Aved, A., and Blasch, E. (2022). Situational
awareness: techniques, challenges, and prospects. AI,
3(1):55–77.
Nguyen, T., Lim, C. P., Nguyen, N. D., Gordon-Brown,
L., and Nahavandi, S. (2019). A review of situation
awareness assessment approaches in aviation environ-
ments. IEEE Systems Journal, 13(3):3590–3603.
Prada, L. R., Mumaw, R. J., Boehm-Davis, D. A., and Boor-
man, D. J. (2006). Testing boeing’s flight deck of the
future: A comparison between current and prototype
autoflight panels. Proceedings of the Human Factors
and Ergonomics Society Annual Meeting, 50(1):55–
58.
Rouwhorst, W., Verhoeven, R., Suijkerbuijk, M., Bos, T.,
Maij, A., Vermaat, M., and Arents, R. (2017). Use
of touch screen display applications for aircraft flight
control. In 2017 IEEE/AIAA 36th Digital Avionics
Systems Conference (DASC), pages 1–10. IEEE.
ICCAS 2024 - International Conference on Cognitive Aircraft Systems
108