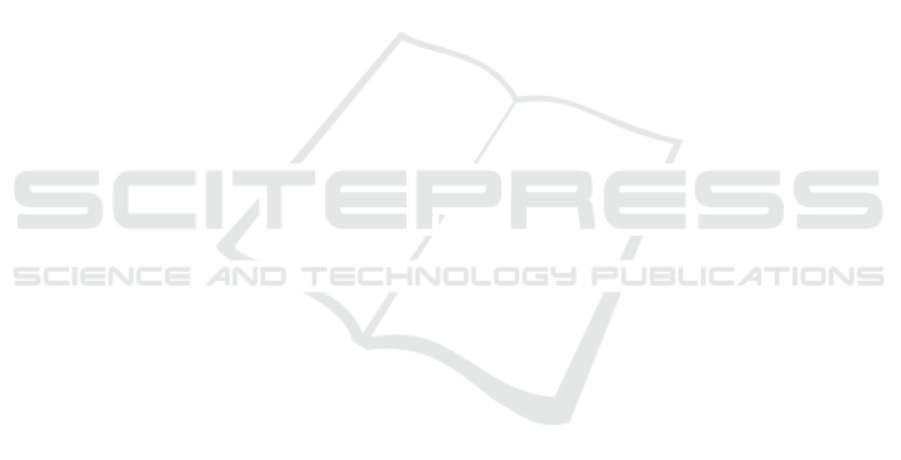
visualizing visitor engagements. However, fully un-
derstanding these engagements poses a broader and
more complex challenge. Future designs could fo-
cus on providing deeper insights into the nature and
nuances of engagement information. With an in-
creasing number of visitors and larger spatial envi-
ronments, hosting becomes an even more challenging
task. Future designs and mechanisms could be devel-
oped to support more efficient and effective hosting
in such complex scenarios. For instance, a prioriti-
zation mechanism based on proximity and the sever-
ity of visitors’ needs could be introduced to enhance
the responsiveness and decision-making capabilities
of the hosts.
We tested the system function by delivering an ex-
emplary Co-MUMR puppet exhibition. A complete
evaluation study of the system’s functionality and the
effectiveness of the visualizations could be conducted
in future work. Additionally, an investigation into bal-
ancing the reduction of visual clutter against the risk
of increased information loss could be conducted to
guide further refinement.
ACKNOWLEDGEMENTS
This project is funded by the European Union and co-
financed from tax revenues on the basis of the budget
adopted by the Saxon State Parliament (Project No.
100690214).
REFERENCES
Cavallo, M. and Forbes, A. G. (2019). CAVE-AR: A VR
authoring system to interactively design, simulate, and
debug multi-user AR experiences. In 2019 IEEE Con-
ference on Virtual Reality and 3D User Interfaces
(VR), pages 872–873. IEEE.
Ens, B. and Irani, P. (2017). Spatial Analytic Inter-
faces: Spatial User Interfaces for In Situ Visual An-
alytics. IEEE Computer Graphics and Applications,
37(2):66–79.
Fleck, P., Calepso, A. S., Hubenschmid, S., Sedlmair, M.,
and Schmalstieg, D. (2023). Ragrug: A toolkit for
situated analytics. IEEE Transactions on Visualization
and Computer Graphics, 29(7):3281–3297.
Guo, Z., Wang, H., Deng, H., Xu, W., Baghaei, N., Lo,
C.-H., and Liang, H.-N. (2024). Breaking the isola-
tion: Exploring the impact of passthrough in shared
spaces on player performance and experience in VR
exergames. pages 1–11. Conference Name: IEEE
Transactions on Visualization and Computer Graph-
ics.
Hou, Z., Xia, Q., Xu, Z., and Tang, Y. (2023). Arradar:
Arrow and radar driven hmd-based visual guidance
in limited field-of-view. In 2023 IEEE International
Symposium on Mixed and Augmented Reality Adjunct
(ISMAR-Adjunct), pages 444–449.
Kalkofen, D., Veas, E., Zollmann, S., Steinberger, M., and
Schmalstieg, D. (2013). Adaptive ghosted views for
augmented reality. In 2013 IEEE International Sym-
posium on Mixed and Augmented Reality (ISMAR),
pages 1–9.
Lin, T., Yang, Y., Beyer, J., and Pfister, H. (2023). Labeling
out-of-view objects in immersive analytics to support
situated visual searching. IEEE Transactions on Visu-
alization and Computer Graphics, 29(3):1831–1844.
Milgram, P., Takemura, H., Utsumi, A., and Kishino, F.
(1995). Augmented reality: a class of displays on the
reality-virtuality continuum. In Das, H., editor, Tele-
manipulator and Telepresence Technologies, volume
2351, pages 282 – 292. International Society for Op-
tics and Photonics, SPIE.
Prouzeau, A., Lhuillier, A., Ens, B., Weiskopf, D., and
Dwyer, T. (2019). Visual Link Routing in Immer-
sive Visualisations. In Proceedings of the 2019 ACM
International Conference on Interactive Surfaces and
Spaces, pages 241–253, Daejeon Republic of Korea.
ACM.
Rowden, A., Krokos, E., Whitley, K., and Varshney, A.
(2023). Exploring effective immersive approaches to
visualizing wifi. In 2023 IEEE International Sym-
posium on Mixed and Augmented Reality (ISMAR),
pages 732–740, Los Alamitos, CA, USA. IEEE Com-
puter Society.
Schier, F., Zeidler, D., Chandran, K., Yu, Z., and McGin-
ity, M. (2023). Viewr: Architectural-scale multi-
user mixed reality with mobile head-mounted dis-
plays. IEEE Transactions on Visualization and Com-
puter Graphics, pages 1–14.
Thanyadit, S., Punpongsanon, P., and Pong, T.-C. (2019).
Observar: Visualization system for observing virtual
reality users using augmented reality. In 2019 IEEE
International Symposium on Mixed and Augmented
Reality (ISMAR), pages 258–268.
Wang, C.-H., Tsai, C.-E., Yong, S., and Chan, L. (2020).
Slice of light: Transparent and integrative transition
among realities in a multi-hmd-user environment. In
Proceedings of the 33rd Annual ACM Symposium on
User Interface Software and Technology, UIST ’20,
page 805–817, New York, NY, USA. Association for
Computing Machinery.
Willett, W., Jansen, Y., and Dragicevic, P. (2017). Embed-
ded data representations. IEEE Transactions on Visu-
alization and Computer Graphics, 23(1):461–470.
Yoo, D., Kantengwa, O., Logler, N., Interayamahanga, R.,
Nkurunziza, J., and Friedman, B. (2018). Collabora-
tive reflection: A practice for enriching research part-
nerships spanning culture, discipline, and time. In
Proceedings of the 2018 CHI Conference on Human
Factors in Computing Systems, CHI ’18, page 1–11,
New York, NY, USA. Association for Computing Ma-
chinery.
Yu, Z., Zeidler, D., Victor, V., and Mcginity, M. (2023). Dy-
nascape: Immersive authoring of real-world dynamic
scenes with spatially tracked rgb-d videos. In Pro-
ceedings of the 29th ACM Symposium on Virtual Re-
ality Software and Technology, VRST ’23, New York,
NY, USA. Association for Computing Machinery.
IVAPP 2025 - 16th International Conference on Information Visualization Theory and Applications
902