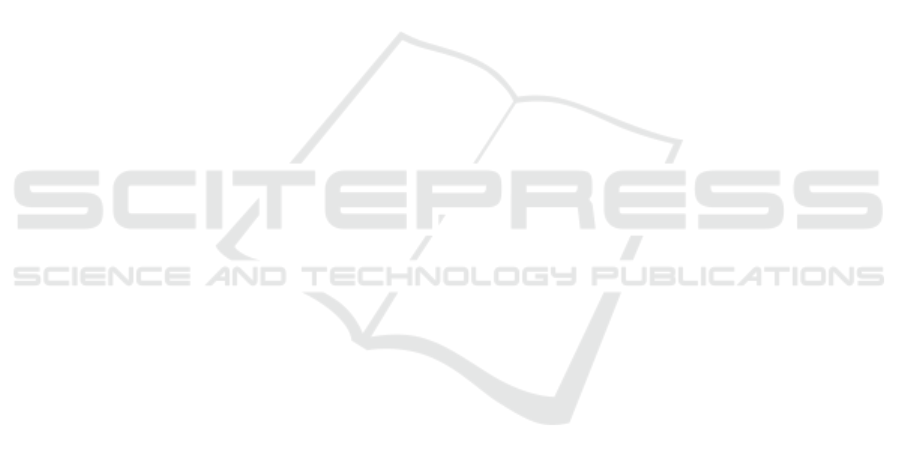
significantly impacting the propulsion system’s per-
formance, was not included in this research project.
Future studies should conduct a comparative anal-
ysis of different controllers, focusing on both cost-
effectiveness and performance. Additionally, the en-
vironmental and economic impact of using end of
life components for the presented mobility solution
should be elaborated quantitatively.
REFERENCES
Ayetor, G., Mbonigaba, I., Sackey, M., and Andoh, P.
(2021). Vehicle regulations in africa: Impact on used
vehicle import and new vehicle sales. Transportation
Research Interdisciplinary Perspectives, 10:100384.
Bako Motors (2024). Website Bako Motors. https://bako
motors.com. Accessed: October 2, 2024.
Cavoli, C. (2021). Accelerating sustainable mobility and
land-use transitions in rapidly growing cities: Identi-
fying common patterns and enabling factors. Journal
of Transport Geography, 94:103093.
Dayang Naki (2024). DY-H6 Hot Selling Africa Cargo
Tricycle Models with Powerful Engine of 250cc.
https://www.dayangnaki.com. Accessed: October 1,
2024.
De Magalh
˜
aes, L. and Santaeul
`
alia-Llopis, R. (2018). The
consumption, income, and wealth of the poorest: An
empirical analysis of economic inequality in rural and
urban sub-saharan africa for macroeconomists. Jour-
nal of Development Economics, 134:350–371.
DW (2018). Durch Autoexporte dicke Luft in Afrika.
https://www.dw.com/de/a-44805459. Accessed: Oc-
tober 11, 2024.
European Union (2022). Could a giant solar array in the
sahara resolve our energy needs? https://cordis.europ
a.eu/article/id/442711. Accessed: October 8, 2024.
ITQ (2024). Bamboo solar car – smart green island projects.
https://stetter-itq.com. Accessed: October 8, 2024.
Izogo, E. E. (2015). Customers’ service quality perception
in automotive repair. African Journal of Economic
and Management Studies, 6(3):272–288.
Kofa (2024). The TAILG Jidi - Powered by Kofa. https:
//www.kofa.co. Accessed: October 7, 2024.
Koroma, B., Oviedo, D., Yusuf, Y., Macarthy, J., Cavoli,
C., Jones, P., Levy, C., and Sellu, S. (2021). City pro-
file: Freetown, base conditions of mobility, accessibil-
ity and land use. T-SUM. UCL.
Luxman Light (2019). The influence of average annual sun-
shine and total solar radiation intensity in africa for so-
lar street light. https://luxmanlight.com/the-influence-
of-average-annual-sunshine. Accessed: October 8,
2024.
Minnerup, K., Kalt, S., Lin, X., Koberstaedt, S., and
Lienkamp, M. (2018). A 48 v drive train for a utility
vehicle designed for rural africa - review and analysis.
In 2018 21st International Conference on Electrical
Machines and Systems (ICEMS), pages 2477–2482.
Moos, M. and Sambo, W. (2018). An exploratory study
of challenges faced by small automotive businesses in
townships : the case of garankuwa, south africa. Jour-
nal of Contemporary Management, 15(1):467–494.
Oviedo, D., Cavoli, C., Yusuf, Y., Koroma, B., and Chong,
A. Z. W. (2024). Everyday accessibility practices
and experiences in a context of transitions to sustain-
able mobility: Qualitative evidence from sub-saharan
africa. International Journal of Sustainable Trans-
portation, 18(4):328–343.
Pojani, D. and Stead, D. (2017). The Urban Transport Cri-
sis in Emerging Economies: An Introduction, pages
1–10. Springer International Publishing, Cham.
Porter, G. (2002). Living in a walking world: Rural mobility
and social equity issues in sub-saharan africa. World
Development, 30(2):285–300.
Porter, G. (2016). Mobilities in rural africa: New connec-
tions, new challenges. Annals of the American Asso-
ciation of Geographers, 106(2):434–441.
QS Motors (2024). QS120 QSD120A-7. https://www.cnqs
motor.com. Accessed: October 12, 2024.
Reichelt Elektronik (2024). Tle4905l. https://www.reichelt
.de. Accessed: August 16, 2024.
Statista (2024a). Afrika: Erzeugung erneuerbarer En-
ergien in Afrika nach Quelle von 2000 bis 2023.
https://de.statista.com. Accessed: October 5, 2024.
Statista (2024b). Afrika: Rangliste der 20 umwelt-
freundlichsten L
¨
ander nach dem Environmen-
tal Performance Index (EPI) im Jahr 2024.
https://de.statista.com. Accessed: September 25,
2024.
Statista (2024c). Average retail prices for gaso-
line in Africa as of February 2024, by country.
https://www.statista.com. Accessed: October 6, 2024.
Statista (2024d). Entwicklung des durchschnittlichen Brut-
toinlandsprodukt (BIP) pro Kopf in Afrika von 2010
bis 2028. https://de.statista.com. Accessed: Septem-
ber 25, 2024.
Statista (2024e). Rangliste der 20 umweltfreundlichsten
L
¨
ander nach dem Environmental Performance Index
(EPI) im Jahr 2024. https://de.statista.com. Accessed:
October 18, 2024.
Teoh, R., Anciaes, P., and Jones, P. (2020). Urban mobility
transitions through gdp growth: Policy choices facing
cities in developing countries. Journal of Transport
Geography, 88:102832.
World Health Organization (2016). Ambient air pollution:
A global assessment of exposure and burden of dis-
ease. Technical report, WHO. Accessed: October 1,
2024.
Zhyt (2024). 1500 W Electric Tricycle Motor 48 V. https:
//www.amazon.de/dp/B0CXXJ5TSD. Accessed:
October 11, 2024.
ˇ
Solt
´
es, M., Koberstaedt, S., Lienkamp, M., Rauchbart, S.,
and Frenkler, F. (2018). Acar - a electric vehicle con-
cept for sub-saharan africa. In 2018 IEEE PES/IAS
PowerAfrica, pages 301–306.
SMARTGREENS 2025 - 14th International Conference on Smart Cities and Green ICT Systems
86