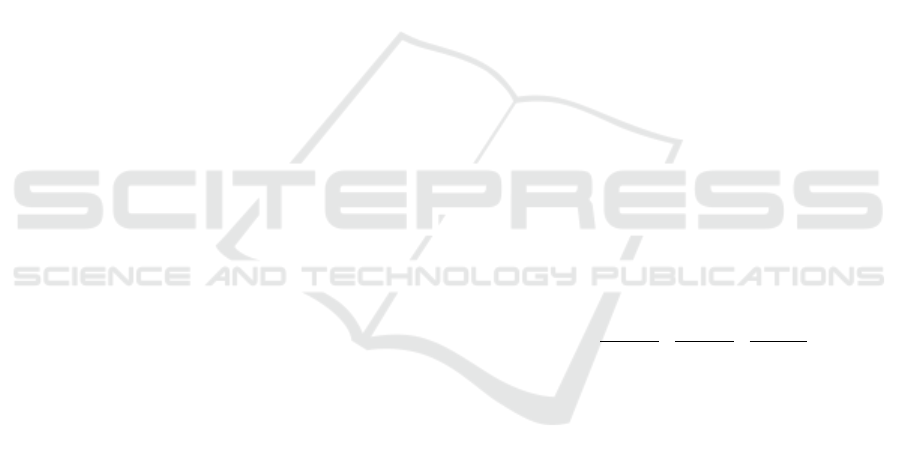
cooling system was suggested for photovoltaic pan-
els to reduce the temperature of the under radiate
area(Mohammadnia et al., 2021).
In the LWPT system, the most important concern
is power transmission efficiency, which is composed
of three parts: electro-optical conversion efficiency of
the laser source, beam’s transmission efficiency in the
free space, and photo-electric conversion efficiency of
the photovoltaic array (PVA). It is well known that at-
mospheric turbulence will severely affect the wave-
front of laser beams(Guo et al., 2022), so that the far-
field intensity distribution of the laser beam changes
constantly, mainly reflected in the beam dithering,
beam spreading, and uneven beam intensity distribu-
tion.
For the PVA in the LWPT link, the size and shape
are specially designed according to the fixed beam
intensity distribution. Turbulence will invalidate this
optimized design, and then reduce the conversion ef-
ficiency of PVA. From the research in recent years,
it can be find that most experiments are carried out
indoors or outdoors at a short distance, so turbu-
lence has a limited effect. In the long-range (7 km)
LWPT experiment, the performance of the LWPT sys-
tem was greatly improved when the turbulence ef-
fect was mitigated(Vorontsov and Weyrauch, 2016).
Many papers have discussed the effect of static beam
intensity distribution (such as Gaussian) on PVA ef-
ficiency(Zhou and Jin, 2017), but the effect of turbu-
lence has not been analyzed yet. As the beam trans-
mission distance of LWPT becomes longer in the fu-
ture, it will be very valuable to study the effects of
turbulence on LWPT.
In this paper, the basic principle of the LWPT sys-
tem is briefly introduced, followed by the problems.
Then the mathematical models, including the turbu-
lence model and PVA model, are introduced, which
can be used to generate varying far-field beam in-
tensity distributions and observe the performance of
PVA. In the end, simulation experiments are con-
ducted to give qualitative conclusions. The experi-
ment results show that the maximum power of PVA
is determined jointly by the mean and uniformity of
the beam intensity. In addition, the moderate turbu-
lence (with C
2
n
of 8.6 × 10
−16
m
−2/3
) can cause a se-
vere power drop of the PVA, and only realizing the tilt
aberration compensation can not effectively alleviate
this phenomenon.
The rest of this paper is organized as follows: the
current situation and problems of LWPT are shown in
section 2. In Section 3, the effects of the atmosphere
turbulence are introduced in detail. In Section 4, sim-
ulation experiments based on turbulence with differ-
ent levels and PVA with different cells are conducted.
2 CURRENT SITUATION AND
PROBLEMS
Fig. 1 shows the structural scheme of the LWPT
system. First, electric power is converted into op-
tical power by the laser source. Then, the optical
power is directed by the acquisition, tracking, and
pointing (ATP) system, and emitted by the optical an-
tenna. Laser beams reach the remoted photovoltaic
array (PVA) after long-range atmospheric transmis-
sion. In the end, the optical power is converted into
electric power by the PVA and then drives the load,
such as a drone.
In the process of free space propagation, the in-
tensity distribution of the laser beam varies randomly
due to the turbulence. The left corner of Fig. 1 shows
the PVA and the laser spot at the target plane in ideal
condition and with turbulence. The intensity distribu-
tion of the spot is generally considered to be Gaussian
distribution in ideal conditions, due to the diffraction
in laser resonators. The shape of the PVA and the
connection of the cells is usually optimized for this
situation, in order to improve the photo-electric con-
version efficiency. When the turbulence is considered,
the spot will deviate from the surface of the PVA, and
the intensity distribution becomes irregular, resulting
in a mismatch with the PVA’s design.
Here, the power transmission efficiency of the en-
tire LWPT link is represented by η, and it can be de-
termined by the following formula:
η = η
T x−io
· η
T x−oRx−i
· η
Rx−io
=
P
T x−out
P
T x−in
·
P
Rx−in
P
T x−out
·
P
Rx−out
P
Rx−in
(1)
The parameter η consists of the following parts:
1)Electro-optical conversion efficiency η
T x−io
,
which equals to the ratio of the emitted optical power
P
T x−out
to the input electrical power P
T x−in
, and it is
mainly determined by the type of the laser.
2)Beam’s transmission efficiency in the free space
η
T x−oRx−i
, which equals to the ratio of the received
optical power P
Rx−in
to the emitted optical power
P
T x−out
, and it is mainly determined by the diffrac-
tion effect, atmospheric absorption, and the occlusion
of obstacles.
3)Photo-electric conversion efficiency η
Rx−io
,
which equals to the ratio of the consumed electrical
power P
Rx−out
to the received optical power P
Rx−in
.
This parameter is determined by the PV materials, the
connection mode of PV cells, the uniformity of the
beam intensity, and the degree of matching between
the shape of the spot and the shape of the PVA. Tur-
bulence will distort the wavefront of the laser beam,
which will lead to the beam dithering, beam spread-
PHOTOPTICS 2025 - 13th International Conference on Photonics, Optics and Laser Technology
42