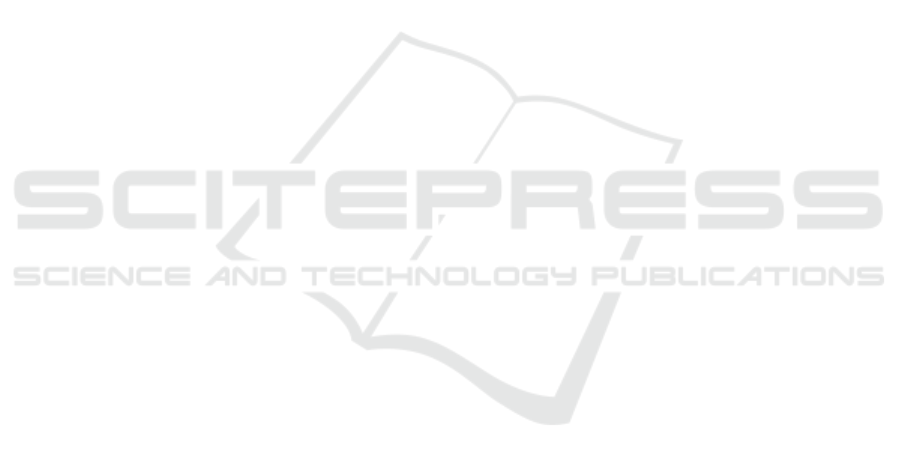
ings of the 6th International Conference on Sustain-
able Information Engineering and Technology, SIET
’21, page 106–112, New York, NY, USA. Association
for Computing Machinery.
Baun, C., Kunze, M., Nimis, J., and Tai, S. (2011).
Cloud Computing - Web-Based Dynamic IT Services.
Springer.
Beckmann, A. (2020). Digitalisierung in der
hochschullehre: Erfahrungen mit dem mathedu
digital-lehrkonzept und zur akzeptanz digitaler
lehrelemente durch die studierenden. Medi-
enP
¨
adagogik: Zeitschrift f
¨
ur Theorie und Praxis
der Medienbildung, page 1–20.
Chen, L., Huang, W., Sui, A., Chen, D., and Sun, C.
(2017). The online education platform using prox-
mox and novnc technology based on laravel frame-
work. In 2017 IEEE/ACIS 16th International Con-
ference on Computer and Information Science (ICIS),
pages 487–491.
Demchenko, Y., Gallenm
¨
uller, S., Fdida, S., Rausch, T., An-
dreou, P., and Saucez, D. (2023). Slices data man-
agement infrastructure for reproducible experimental
research on digital technologies. In 2023 IEEE Globe-
com Workshops (GC Wkshps), pages 1–6.
Dietz, M. (2023). The internet of digital twins: Ad-
vances in hyperscaling virtual labs with hypervisor-
and container-based virtualization. In Auer, M. E.,
Pachatz, W., and R
¨
u
¨
utmann, T., editors, Learning in
the Age of Digital and Green Transition, pages 574–
586, Cham. Springer International Publishing.
Emiliano, R. and Antunes, M. (2015). Automatic network
configuration in virtualized environment using gns3.
In 2015 10th International Conference on Computer
Science & Education (ICCSE), pages 25–30.
Erpenbeck, J. and Sauter, W. (2017). Handbuch Kompeten-
zentwicklung im Netz Bausteine einer neuen Lernwelt.
Sch
¨
affer-Poeschel, Stuttgart, Germany, 1 edition.
Handke, J. (2015). Handbuch Hochschullehre Digital. Tec-
tum Wissenschaftsverlag, Marburg an der Lahn, Ger-
many, 1 edition.
Handke, J. and Sch
¨
afer, A. M. (2012). E-Learning, E-
Teaching und E-Assessment in der Hochschullehre.
Oldenbourg Wissenschaftsverlag Verlag, M
¨
unchen.
Kappes, M. (2023). Netzwerk- und Datensicherheit.
Springer Vieweg, Wiesbaden, Germany, 3 edition.
Lantz, B., Heller, B., and McKeown, N. (2010). A network
in a laptop: rapid prototyping for software-defined
networks. In Proceedings of the 9th ACM SIGCOMM
Workshop on Hot Topics in Networks, Hotnets-IX,
New York, NY, USA. Association for Computing Ma-
chinery.
Mahalingam, M., Dutt, D., Duda, K., Agarwal, P., Kreeger,
L., Sridhar, T., Bursell, M., and Wright, C. (2014).
Virtual eXtensible Local Area Network (VXLAN): A
Framework for Overlaying Virtualized Layer 2 Net-
works over Layer 3 Networks. RFC 7348.
Maler, E., Machulak, M., Richer, J., and Hardjono, T.
(2019). Federated Authorization for User-Managed
Access (UMA) 2.0. Internet-Draft draft-maler-oauth-
umafedauthz-00, Internet Engineering Task Force.
Work in Progress.
Meneses, S., Maya, E., and Vasquez, C. (2021). Network
design defined by software on a hyper-converged in-
frastructure. case study: Northern technical univer-
sity fica data center. In Botto-Tobar, M., Zamora,
W., Larrea Pl
´
ua, J., Bazurto Roldan, J., and Santa-
mar
´
ıa Philco, A., editors, Systems and Information
Sciences, pages 272–280, Cham. Springer Interna-
tional Publishing.
Richards, M. and Ford, N. (2020). Fundamentals of soft-
ware architecture. O’Reilly Media, Sebastopol, CA.
Serrano, M., Isaris, N., and Schaffers, H., editors (2022).
Building the future internet through FIRE. Taylor &
Francis, London, England.
Steffen Auer, A.-F.-S. E. (2024). linuxmuster.net
– documentation. accessed March 10, 2025,
https://www.linuxmuster.net/en/documentation/.
Thakkar, M. (2020). Building React Apps with server-Side
Rendering: Use React, redux, and Next to build full
server-Side Rendering applications. APRESS, New
York, NY.
Wolf, W. (2009). Cyber-physical systems. Computer,
42(3):88–89.
The Virtual Computer Networks Lab: On the Design and Implementation of a Location Independent Networks Laboratory in Higher
Education
207