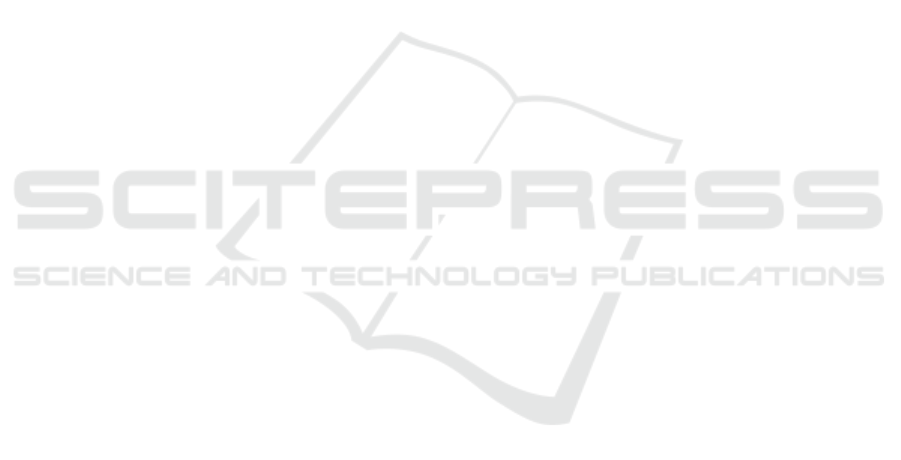
ACKNOWLEDGEMENTS
This research is funded by University of Informa-
tion Technology-Vietnam National University Ho Chi
Minh City under grant number D1-2024-30.
REFERENCES
Alammary, A., Alhazmi, S., Almasri, M., and Gillani, S.
(2019). Blockchain-based applications in education:
A systematic review. Applied Sciences, 9(12):2400.
Benet, J. (2014). IPFS-Content Addressed, Versioned, P2P
File System (DRAFT 3). Technical report.
Brandst
¨
atter, T., Schulte, S., Cito, J., and Borkowski, M.
(2020). Characterizing efficiency optimizations in so-
lidity smart contracts. In 2020 IEEE International
Conference on Blockchain (Blockchain), pages 281–
290. IEEE.
Buterin, V. et al. (2014). A next-generation smart contract
and decentralized application platform. white paper,
3(37):2–1.
Daniel, E. and Tschorsch, F. (2022). Ipfs and friends: A
qualitative comparison of next generation peer-to-peer
data networks. IEEE Communications Surveys & Tu-
torials, 24(1):31–52.
Feist, J., Grieco, G., and Groce, A. (2019). Slither: a
static analysis framework for smart contracts. In 2019
IEEE/ACM 2nd International Workshop on Emerging
Trends in Software Engineering for Blockchain (WET-
SEB), pages 8–15. IEEE.
Han, M., Li, Z., He, J., Wu, D., Xie, Y., and Baba, A.
(2018). A novel blockchain-based education records
verification solution. In Proceedings of the 19th an-
nual SIG conference on information technology edu-
cation, pages 178–183.
Islam, M. A. and Shuvo, S. A. (2024). Blockchain tech-
nology: a tool to solve the challenges of the education
sector in developing countries. International Journal
of Computational Systems Engineering, 8(1-2):75–86.
Jiang, S., Jakobsen, K., Bueie, J., Li, J., and Haro, P. H.
(2022). A tertiary review on blockchain and sustain-
ability with focus on sustainable development goals.
IEEE access, 10:114975–115006.
Lavaur, T., Detchart, J., Lacan, J., and Chanel, C. P. (2023).
Modular zk-rollup on-demand. Journal of Network
and Computer Applications, 217.
Ma, Y. and Fang, Y. (2020). Current status, issues, and chal-
lenges of blockchain applications in education. Inter-
national Journal of Emerging Technologies in Learn-
ing (IJET), 15(12):20–31.
Metcalfe, W. et al. (2020). Ethereum, smart contracts,
dapps. Blockchain and Crypt Currency, 77:77–93.
Nagele, J. and Schett, M. A. (2020). Blockchain superopti-
mizer. arXiv preprint arXiv:2005.05912.
Patil, K. et al. (2021). Usability of blockchain technology
in higher education: A systematic review identifying
the current issues in the education system. In Jour-
nal of Physics: Conference Series, volume 1964, page
042017. IOP Publishing.
Rahman, T., Mouno, S. I., Raatul, A. M., Al Azad, A. K.,
and Mansoor, N. (2023). Verifi-chain: a creden-
tials verifier using blockchain and ipfs. In Inter-
national Conference on Information, Communication
and Computing Technology, pages 361–371. Springer.
Rustemi, A., Dalipi, F., Atanasovski, V., and Risteski, A.
(2023). A systematic literature review on blockchain-
based systems for academic certificate verification.
IEEE Access, 11:64679–64696.
Shi, X., Xiao, H., Liu, W., Lackner, K. S., Buterin, V.,
and Stocker, T. F. (2023). Confronting the carbon-
footprint challenge of blockchain. Environmental sci-
ence & technology, 57(3):1403–1410.
Smith, N. M., Hoal, K. E. O., and Thompson, J. F. (2020).
Ensure inclusive and equitable quality education and
promote lifelong learning opportunities for all. In
Mining, materials, and the sustainable development
goals (SDGs), pages 29–38. CRC Press.
Studiorum, A. M. and Donno, L. (2022). Optimistic and
Validity Rollups: Analysis and Comparison between
Optimism and StarkNet. Technical report.
Thibault, L. T., Sarry, T., and Hafid, A. (2022). Blockchain
scaling using rollups: A comprehensive survey.
Institute of Electrical and Electronics Engineers,
10:93039–93054.
V
´
azquez-Ingelmo, A., Garc
´
ıa-Holgado, A., and Garc
´
ıa-
Pe
˜
nalvo, F. J. (2020). C4 model in a software en-
gineering subject to ease the comprehension of uml
and the software. In 2020 IEEE Global Engineering
Education Conference (EDUCON), pages 919–924.
IEEE.
Voicu-Dorobantu, R., Udokwu, C., and Bocse, B. (2021).
Using blockchain for optimal and transparent resource
allocation: A proposed solution for fund allocation:
Brief overview. In Proceedings of the 5th Interna-
tional Conference on E-Commerce, E-Business and E-
Government, pages 35–38.
Zhou, Y., Luo, X., and Zhou, M. (2023). Cryptocurrency
transaction network embedding from static and dy-
namic perspectives: An overview. IEEE/CAA Journal
of Automatica Sinica, 10(5):1105–1121.
Blockchain Solutions for Scalable and Sustainable Education: Enhancing Credentialing and Resource Management
223