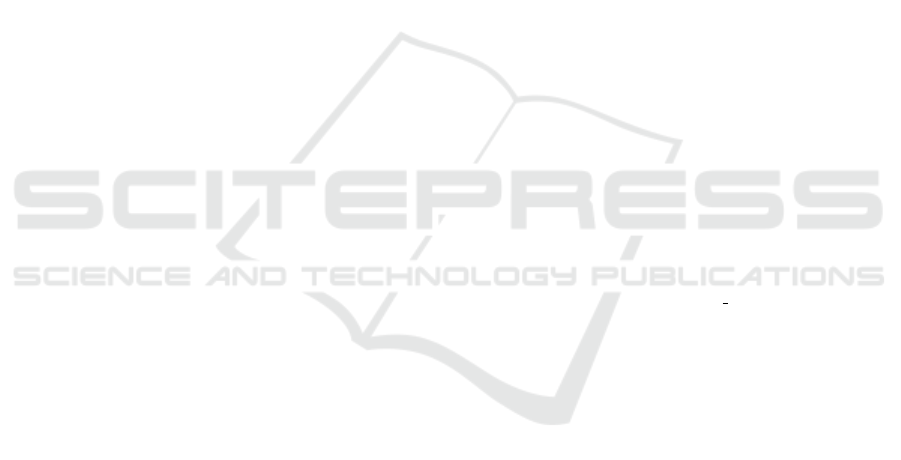
REFERENCES
Ashton, K. et al. (2009). That ‘internet of things’ thing.
RFID journal, 22(7):97–114.
Atzori, L., Iera, A., and Morabito, G. (2010). The internet of
things: A survey. Computer networks, 54(15):2787–
2805.
Augustin, A., Yi, J., Clausen, T., and Townsley, W. M.
(2016). A study of LoRa: Long range & low
power networks for the Internet of Things. Sensors,
16(9):1466.
Aung, M. M. and Chang, Y. S. (2014). Temperature man-
agement for the quality assurance of a perishable food
supply chain. Food Control, 40:198–207.
Badia-Melis, R., Mc Carthy, U., Ruiz-Garcia, L., Garcia-
Hierro, J., and Villalba, J. R. (2018). New trends in
cold chain monitoring applications - A review. Food
Control, 86:170–182.
Bose, A., Aithal, A. K., and Rajeshwari, B. (2022). Vac-
cine cold storage monitoring and tracking using Lo-
RaWAN. In 2022 2nd International Conference on
Intelligent Technologies (CONIT), pages 1–6. IEEE.
Cattani, M., Boano, C. A., and R
¨
omer, K. (2017). An ex-
perimental evaluation of the reliability of LoRa long-
range low-power wireless communication. Journal of
Sensor and Actuator Networks, 6(2):7.
Cavalcante, A. M., Marquezini, M. V., Mendes, L.,
and Moreno, C. S. (2021). 5G for remote areas:
Challenges, opportunities and business modeling for
Brazil. IEEE Access, 9:10829–10843.
Centenaro, M., Vangelista, L., Zanella, A., and Zorzi, M.
(2016). Long-range communications in unlicensed
bands: The rising stars in the IoT and smart city sce-
narios. IEEE Wireless Communications, 23(5):60–67.
EMQ Technologies Inc (2024). Free public MQTT Bro-
ker. https://www.emqx.com/en/mqtt/public-mqtt5-
broker. Accessed: 2024-06-20.
Enriko, I., Alemuda, F., and Adrianto, D. (2022). An Ap-
proach to Monitor Vaccine Quality During Distribu-
tion Using Internet of Things. Journal of Information
Science & Engineering, 38(5).
Fan, H. W. and Monteiro, W. M. (2018). History and per-
spectives on how to ensure antivenom accessibility in
the most remote areas in Brazil. Toxicon, 151:15–23.
Fargas, B. C. and Petersen, M. N. (2017). GPS-free ge-
olocation using LoRa in low-power WANs. In 2017
global internet of things summit (Giots), pages 1–6.
IEEE.
Frangoudis, P. A., Tsigkanos, C., and Dustdar, S. (2021).
Connectivity technology selection and deployment
strategies for IoT service provision over LPWAN.
IEEE Internet Computing, 25(1):61–70.
Garcia, A. F. and de Souza, W. L. (2023). Internet of
Things Applications for Cold Chain Vaccine Track-
ing: A Systematic Literature Review. In International
Conference on Information Technology-New Genera-
tions, pages 323–330. Springer.
Google Maps Platform (2024). Geolocation API Overview.
https://developers.google.com/maps/documentation/
geolocation/overview. Accessed: 2024-06-20.
Jennings, C., Shelby, Z., Arkko, J., Keranen, A., and Bor-
mann, C. (2018). Sensor measurement lists (SenML).
Technical report, Internet Engineering Task Force
(IETF).
KPN (2024a). KPN Things Developer Manual - API
Access. https://docs.kpnthings.com/dm/concepts/api.
Accessed: 2024-06-20.
KPN (2024b). KPN Things Explorer. https:
//www.kpn.com/zakelijk/internet-of-things/kpn-
things/explorer.htm. Accessed: 2024-06-20.
KPN (2024c). KPN Things LoRa Geolocation. https://
docs.kpnthings.com/lora/geolocation/intro. Accessed:
2024-06-20.
Labdaoui, N., Nouvel, F., and Dutertre, S. (2023). Energy-
efficient IoT Communications: A Comparative Study
of Long-Term Evolution for Machines (LTE-M) and
Narrowband Internet of Things (NB-IoT) Technolo-
gies. In 2023 IEEE Symposium on Computers and
Communications (ISCC), pages 823–830. IEEE Com-
puter Society.
Lawrence, P. W., Phippard, T. M., Ramachandran, G. S.,
and Hughes, D. (2018). Developing the IoT to sup-
port the health sector: A case study from Kikwit,
DR Congo. In Emerging Technologies for Develop-
ing Countries: First International EAI Conference,
AFRICATEK 2017, Marrakech, Morocco, March 27-
28, 2017 Proceedings 1st, pages 45–56. Springer.
LoRa Alliance (2024). LoRaWAN Network Coverage
- LoRa Alliance. https://lora-alliance.org/lorawan-
network-coverage. Accessed: 2024-06-20.
Mishra, B. and Kertesz, A. (2020). The use of MQTT
in M2M and IoT systems: A survey. Ieee Access,
8:201071–201086.
Roger Coud
´
e VE2DBE (2024). Radio Mobile Online. https:
//www.ve2dbe.com/rmonline
s.asp. Accessed: 2024-
06-20.
Saban, M., Aghzout, O., Medus, L. D., and Rosado, A.
(2021). Experimental analysis of IoT networks based
on LoRa/LoRaWAN under indoor and outdoor en-
vironments: Performance and limitations. IFAC-
PapersOnLine, 54(4):159–164.
The Things Industries (2024). The Things Stack HTTP
(REST) API. https://www.thethingsindustries.com/
docs/api/reference/http/routes/. Accessed: 2024-06-
20.
Vangelista, L., Zanella, A., and Zorzi, M. (2015). Long-
range IoT technologies: The dawn of LoRa™. In
Future Access Enablers for Ubiquitous and Intelli-
gent Infrastructures: First International Conference,
FABULOUS 2015, Ohrid, Republic of Macedonia,
September 23-25, 2015, pages 51–58. Springer.
Wieringa, R. J. (2014). Design science methodology
for information systems and software engineering.
Springer.
Zinas, N., Kontogiannis, S., Kokkonis, G., Valsamidis, S.,
and Kazanidis, I. (2017). Proposed open source ar-
chitecture for Long Range monitoring. the case study
of cattle tracking at Pogoniani. In Proceedings of the
21st Pan-Hellenic Conference on Informatics, pages
1–6.
ICEIS 2025 - 27th International Conference on Enterprise Information Systems
1060