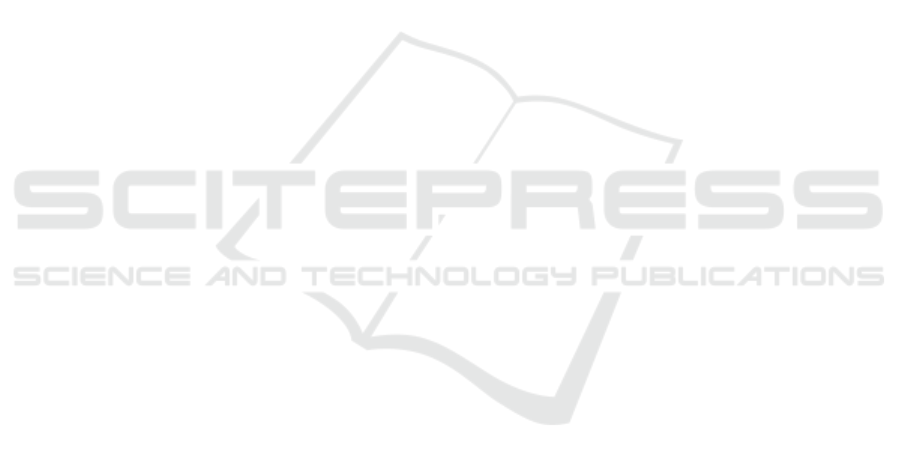
Hoy, M. M. P., Egan, M. Y., and Feder, K. P. (2011). A
Systematic Review of Interventions to Improve Hand-
writing. Canadian Journal of Occupational Therapy,
78(1):13–25. Publisher: SAGE Publications Inc.
James, K. H. and Engelhardt, L. (2012). The effects of
handwriting experience on functional brain develop-
ment in pre-literate children. Trends in Neuroscience
and Education, 1(1):32–42.
Kiefer, M. and Velay, J.-L. (2016). Writing in the digital
age. Trends in Neuroscience and Education, 5(3):77–
81.
Lee, Y., Limbu, B., Rusak, Z., and Specht, M. (2023).
Role of Multimodal Learning Systems in Technology-
Enhanced Learning (TEL): A Scoping Review. In
Viberg, O., Jivet, I., Mu
˜
noz-Merino, P. J., Perifanou,
M., and Papathoma, T., editors, Responsive and Sus-
tainable Educational Futures, pages 164–182, Cham.
Springer Nature Switzerland.
Li, D., Wang, D., Zou, J., Li, C., Qian, H., Yan, J., and He,
Y. (2023). Effect of physical activity interventions on
children’s academic performance: a systematic review
and meta-analysis. European Journal of Pediatrics,
182(8):3587–3601.
Limbu, B. and Chounta, I.-A. (2025). Dataset for the paper
”can the mighty pen be mightier? investigating the
role of haptic senses in multimodal immersive learn-
ing environments”.
Limbu, B., van Helden, G., Barnes, J., and Specht, M.
(2022). We can teach more than we can tell: com-
bining Deliberate Practice, Embodied Cognition, and
Multimodal Learning, volume 3247 of CEUR Work-
shop proceedings, pages 15–21. CEUR-WS.
Limbu, B. H., Jarodzka, H., Klemke, R., and Specht, M.
(2018). Using sensors and augmented reality to train
apprentices using recorded expert performance: A
systematic literature review. Educational Research
Review, 25(June 2017):1–22. Publisher: Elsevier.
Limbu, B. H., Jarodzka, H., Klemke, R., and Specht, M.
(2019). Can You Ink While You Blink? Assessing
Mental Effort in a Sensor-Based Calligraphy Trainer.
Sensors, 19(14):3244. Number: 14 Publisher: Multi-
disciplinary Digital Publishing Institute.
Longcamp, M., Zerbato-Poudou, M.-T., and Velay, J.-L.
(2005). The influence of writing practice on letter
recognition in preschool children: A comparison be-
tween handwriting and typing. Acta Psychologica,
119(1):67–79.
Loup-Escande, E., Frenoy, R., Poplimont, G., Thouvenin,
I., Gapenne, O., and Megalakaki, O. (2017). Contri-
butions of mixed reality in a calligraphy learning task:
Effects of supplementary visual feedback and exper-
tise on cognitive load, user experience and gestural
performance. Computers in Human Behavior, 75:42–
49.
Marucci, M., Di Flumeri, G., Borghini, G., Sciaraffa, N.,
Scandola, M., Pavone, E. F., Babiloni, F., Betti, V.,
and Aric
`
o, P. (2021). The impact of multisensory in-
tegration and perceptual load in virtual reality settings
on performance, workload and presence. Scientific
Reports, 11(1):4831. Publisher: Nature Publishing
Group.
Mat Sanusi, K. A., Mitri, D. D., Limbu, B., and Klemke, R.
(2021). Table Tennis Tutor: Forehand Strokes Clas-
sification Based on Multimodal Data and Neural Net-
works. Sensors, 21(9):3121. Number: 9 Publisher:
Multidisciplinary Digital Publishing Institute.
Mayer, R. E. (2005). Cognitive Theory of Multimedia
Learning, page 31–48. Cambridge Handbooks in Psy-
chology. Cambridge University Press.
McCarroll, H. and Fletcher, T. (2017). Does handwriting in-
struction have a place in the instructional day? The re-
lationship between handwriting quality and academic
success. Cogent Education, 4(1):1386427.
Meik, A., Schneider, J., and Schiffner, D. (2021). Get your
back straight! learn pilates with the pilates correction
app. In DELFI 2021, pages 163–168. Gesellschaft f
¨
ur
Informatik e.V., Bonn.
Mueller, P. A. and Oppenheimer, D. M. (2014). The Pen
Is Mightier Than the Keyboard: Advantages of Long-
hand Over Laptop Note Taking. Psychological Sci-
ence, 25(6):1159–1168. Publisher: SAGE Publica-
tions Inc.
Ose Askvik, E., van der Weel, F. R. R., and van der Meer,
A. L. H. (2020). The Importance of Cursive Handwrit-
ing Over Typewriting for Learning in the Classroom:
A High-Density EEG Study of 12-Year-Old Children
and Young Adults. Frontiers in Psychology, 11. Pub-
lisher: Frontiers.
Paul A. Kirschner, J. S. and Clark, R. E. (2006). Why
minimal guidance during instruction does not work:
An analysis of the failure of constructivist, discovery,
problem-based, experiential, and inquiry-based teach-
ing. Educational Psychologist, 41(2):75–86.
Peverly, S. T. (2006). The Importance of Handwriting
Speed in Adult Writing. Developmental Neuropsy-
chology, 29(1):197–216.
Ray, K., Dally, K., Rowlandson, L., Tam, K. I., and Lane,
A. E. (2022). The relationship of handwriting abil-
ity and literacy in kindergarten: a systematic re-
view. Reading and Writing, 35:11191155. Publisher:
Springer Science and Business Media B.V.
Schneider, J., Romano, G., and Drachsler, H. (2019). Be-
yond Reality—Extending a Presentation Trainer with
an Immersive VR Module. Sensors, 19(16):3457.
Number: 16 Publisher: Multidisciplinary Digital Pub-
lishing Institute.
Shvarts, A. and van Helden, G. (2023). Embodied learn-
ing at a distance: from sensory-motor experience to
constructing and understanding a sine graph. Mathe-
matical Thinking and Learning, 25(4):409–437.
Skar, G. B., Lei, P.-W., Graham, S., Aasen, A. J., Johansen,
M. B., and Kvistad, A. H. (2022). Handwriting flu-
ency and the quality of primary grade students’ writ-
ing. Reading and Writing, 35(2):509–538.
Smoker, T. J., Murphy, C. E., and Rockwell, A. K. (2009).
Comparing Memory for Handwriting versus Typing.
Proceedings of the Human Factors and Ergonomics
Society Annual Meeting, 53(22):1744–1747. Pub-
lisher: SAGE Publications Inc.
Specht, M., Hang, L. B., and Barnes, J. S. (2019). Sen-
Can the Mighty Pen Be Mightier? Investigating the Role of Haptic Senses in Multimodal Immersive Learning Environments
81