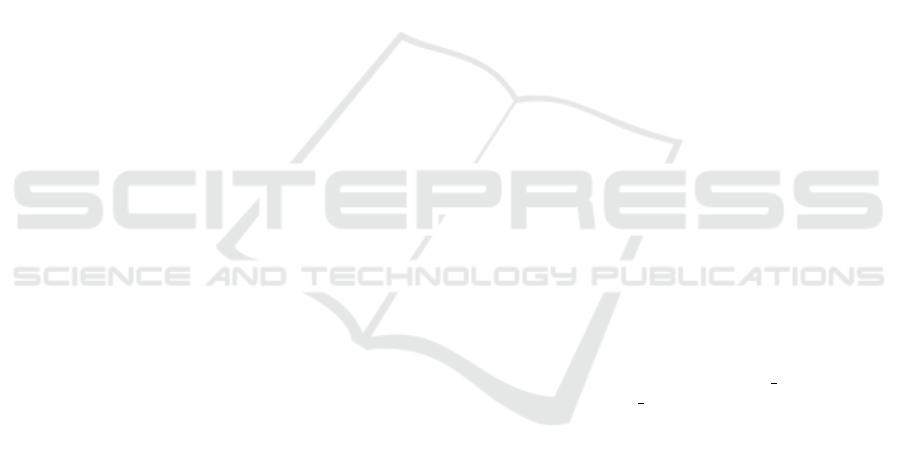
reality sickness questionnaire (VRSQ): Motion sickness
measurement index in a virtual reality environment. Ap-
plied Ergonomics, 69:66–73.
Kloiber, S., Settgast, V., Schinko, C., Weinzerl, M., Fritz, J.,
Schreck, T., and Preiner, R. (2020). Immersive analysis
of user motion in VR applications. The Visual Computer,
36(10-12):1937–1949.
Kr
¨
osl, K., Bauer, D., Schw
¨
arzler, M., Fuchs, H., Suter, G.,
and Wimmer, M. (2018). A VR-based user study on the
effects of vision impairments on recognition distances of
escape-route signs in buildings. The Visual Computer,
34:911–923.
Langbehn, E., Eichler, T., Ghose, S., von Luck, K., Bruder,
G., and Steinicke, F. (2015). Evaluation of an omni-
directional walking-in-place user interface with virtual
locomotion speed scaled by forward leaning angle. In
Proceedings of the GI Workshop on Virtual and Aug-
mented Reality (GI VR/AR), pages 149–160, Sankt Au-
gustin, Germany. GI-Group VR/AR.
Langbehn, E., Lubos, P., Bruder, G., and Steinicke, F.
(2017). Application of redirected walking in room-scale
VR. In 2017 IEEE Virtual Reality (VR), pages 449–450.
Langbehn, E., Lubos, P., and Steinicke, F. (2018). Eval-
uation of Locomotion Techniques for Room-Scale VR:
Joystick, Teleportation, and Redirected Walking. In Pro-
ceedings of the Virtual Reality International Conference
- Laval Virtual, VRIC ’18, New York, NY, USA. Associ-
ation for Computing Machinery.
Majetich, J. (2021). VR Wheelchair. https://github.com/
justinmajetich/vr-wheelchair.
Mori, S., Hashiguchi, S., Shibata, F., and Kimura, A.
(2023). Point & teleport with orientation specification,
revisited: Is natural turning always superior? Journal of
Information Processing, 31:392–403.
Mott, M., Cutrell, E., Gonzalez Franco, M., Holz, C., Ofek,
E., Stoakley, R., and Ringel Morris, M. (2019). Ac-
cessible by Design: An Opportunity for Virtual Real-
ity. In 2019 IEEE International Symposium on Mixed
and Augmented Reality Adjunct (ISMAR-Adjunct), pages
451–454, Beijing, China. IEEE.
Mott, M., Tang, J., Kane, S., Cutrell, E., and Ringel Mor-
ris, M. (2020). “I Just Went into It Assuming That I
Wouldn’t Be Able to Have the Full Experience”: Under-
standing the Accessibility of Virtual Reality for People
with Limited Mobility. In The 22nd International ACM
SIGACCESS Conference on Computers and Accessibil-
ity, ASSETS ’20, New York, NY, USA. Association for
Computing Machinery.
Ohshima, T., Ishihara, H., and Shibata, R. (2016). Virtual
ISU: Locomotion Interface for Immersive VR Gaming
in Seated Position. In Proceedings of the 2016 Virtual
Reality International Conference, VRIC ’16, New York,
NY, USA. Association for Computing Machinery.
Sarupuri, B., Hoermann, S., Steinicke, F., and Lindeman,
R. W. (2017). Triggerwalking: A Biomechanically-
Inspired Locomotion User Interface for Efficient Realis-
tic Virtual Walking. In Proceedings of the 5th Symposium
on Spatial User Interaction, SUI ’17, page 138–147,
New York, NY, USA. Association for Computing Ma-
chinery.
Soccini, A. M. (2020). The induced finger movements ef-
fect. In SIGGRAPH Asia 2020, pages 1–2, New York,
NY, USA. ACM.
Soccini, A. M. and Cena, F. (2021). The ethics of rehabili-
tation in virtual reality: the role of self-avatars and deep
learning. In 2021 IEEE International Conference on
Artificial Intelligence and Virtual Reality (AIVR), pages
324–328, New York, NY, USA. IEEE, IEEE.
Soccini, A. M., Clocchiatti, A., and Inamura, T. (2022). Ef-
fects of frequent changes in extended self-avatar move-
ments on adaptation performance. Journal of Robotics
and Mechatronics, 34(4):756–766.
Vailland, G., Devigne, L., Pasteau, F., Nouviale, F., Fraudet,
B., Leblong,
´
E., Babel, M., and Gouranton, V. (2021).
VR based Power Wheelchair Simulator: Usability Eval-
uation through a Clinically Validated Task with Regular
Users. In 2021 IEEE Virtual Reality and 3D User Inter-
faces (VR), pages 420–427, Lisboa, Portugal. IEEE.
Weißker, T., Kunert, A., Fr
¨
ohlich, B., and Kulik, A. (2018).
Spatial Updating and Simulator Sickness During Steer-
ing and Jumping in Immersive Virtual Environments. In
2018 IEEE Conference on Virtual Reality and 3D User
Interfaces (VR), pages 97–104, Lisboa, Portugal. IEEE.
Wilson, P. T., Kalescky, W., MacLaughlin, A., and
Williams, B. (2016). VR Locomotion: Walking > Walk-
ing in Place > Arm Swinging. In Proceedings of the 15th
ACM SIGGRAPH Conference on Virtual-Reality Contin-
uum and Its Applications in Industry - Volume 1, VRCAI
’16, page 243–249, New York, NY, USA. Association for
Computing Machinery.
Wolf, D., Rogers, K., Kunder, C., and Rukzio, E. (2020).
JumpVR: Jump-Based Locomotion Augmentation for Vir-
tual Reality, page 1–12. Association for Computing Ma-
chinery, New York, NY, USA.
World Health Organization (WHO) (2017). Wheelchair
publications – an overview. https://www.who.int/
news-room/articles-detail/wheelchair\ publications\
%E2\%80\%93an\ overview.
World Health Organization (WHO) (2021). Disability
and health. https://www.who.int/news-room/fact-sheets/
detail/disability-and-health.
Yang, Y.-S., Koontz, A. M., Hsiao, Y.-H., Pan, C.-T., and
Chang, J.-J. (2021). Assessment of Wheelchair Propul-
sion Performance in an Immersive Virtual Reality Sim-
ulator. International Journal of Environmental Research
and Public Health, 18(15):8016.
Zielasko, D. and Riecke, B. E. (2020). Can We Give Seated
Users in Virtual Reality the Sensation of Standing or
Even Walking? Do We Want To? In 2020 IEEE Con-
ference on Virtual Reality and 3D User Interfaces Ab-
stracts and Workshops (VRW), pages 281–282, Atlanta,
GA, USA. IEEE.
Exploring Seated Locomotion Techniques in Virtual Reality for People with Limited Mobility
173