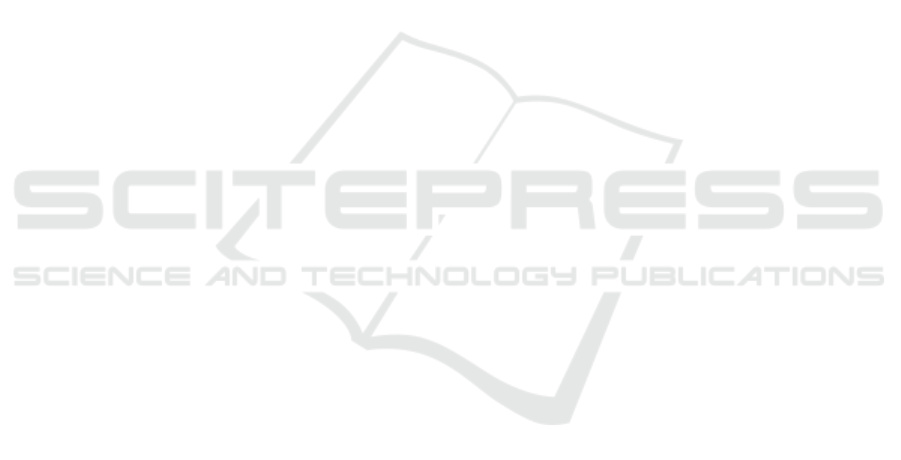
Hussey, N. E., Kessel, S. T., Aarestrup, K., Cooke, S. J.,
Cowley, P. D., Fisk, A. T., Harcourt, R. G., Holland,
K. N., Iverson, S. J., Kocik, J. F., et al. (2015). Aquatic
animal telemetry: a panoramic window into the under-
water world. Science, 348(6240):1255642.
Judge, P. (2021). Prince william county environmental
report [...]. https://www.datacenterdynamics.com/
en/news/prince-william-county-environment-report-
recommend-ditching-2000-acre-data-center-plans/.
Accessed: 2024-12-25.
Kays, R., Crofoot, M. C., Jetz, W., and Wikelski, M. (2015).
Terrestrial animal tracking as an eye on life and planet.
Science, 348(6240):aaa2478.
Kshetri, N. and Voas, J. (2024). Data centers in your back-
yard? Computer, 57(1):82–87.
Lamarche-Beauchesne, R. (2023). Reviving the silenced;
defining vegan fashion and classifying materials of an-
imal origin. Fashion Theory, 27(7):937–956.
Liu, J.-T., Hammitt, J. K., and Wang, J.-L. (2022). Revis-
iting the value of a statistical life: an international ap-
proach. Journal of Benefit-Cost Analysis, 13(1):123–
146.
Lohmann, P. M., Gsottbauer, E., Farrington, J., Human,
S., and Reisch, L. A. (2024). Choice architecture
promotes sustainable choices in online food-delivery
apps. PNAS nexus, 3(10):pgae422.
Low, P., Panksepp, J., Reiss, D., Edelman, D.,
Van Swinderen, B., and Koch, C. (2012). The cam-
bridge declaration on consciousness. In Francis crick
memorial conference, volume 7. Cambridge England.
McKinney, M. L. (2008). Effects of urbanization on species
richness: a review of plants and animals. Urban
ecosystems, 11:161–176.
Morton, D. B. (2023). A model framework for the esti-
mation of animal ‘suffering’: Its use in predicting and
retrospectively assessing the impact of experiments on
animals. Animals, 13(5):800.
Neethirajan, S. (2021). Ethics of digital animal farming.
Preprints.
Neethirajan, S. (2023). The significance and ethics of digital
livestock farming. AgriEngineering, 5(1):488–505.
Pistollato, F. and Battino, M. (2014). Role of plant-based di-
ets in the prevention and regression of metabolic syn-
drome and neurodegenerative diseases. Trends in food
science & technology, 40(1):62–81.
Rabelo, A. C., Oliveira, I. L., and Lisboa-Filho, J. (2017).
An architectural model for smart cities using collab-
orative spatial data infrastructures. In International
Conference on Smart Cities and Green ICT Systems,
volume 2, pages 242–249. SCITEPRESS.
Rebolo-Ifr
´
an, N., Grilli, M. G., and Lambertucci, S. A.
(2019). Drones as a threat to wildlife: Youtube com-
plements science in providing evidence about their ef-
fect. Environmental Conservation, 46(3):205–210.
Rodger, D. (2022). Why we should stop using animal-
derived products on patients without their consent.
Journal of medical ethics, 48(10):702–706.
Ruini, L. F., Ciati, R., Pratesi, C. A., Marino, M., Princi-
pato, L., and Vannuzzi, E. (2015). Working toward
healthy and sustainable diets: The “double pyramid
model” developed by the barilla center for food and
nutrition to raise awareness about the environmental
and nutritional impact of foods. Frontiers in nutrition,
2:126479.
Satija, A. and Hu, F. B. (2018). Plant-based diets
and cardiovascular health. Trends in cardiovascular
medicine, 28(7):437–441.
Scarborough, P., Clark, M., Cobiac, L., Papier, K., Knup-
pel, A., Lynch, J., Harrington, R., Key, T., and Spring-
mann, M. (2023). Vegans, vegetarians, fish-eaters and
meat-eaters in the uk show discrepant environmental
impacts. Nature Food, 4(7):565–574.
Schelling, T. C. (1968). The life you save may be your own.
In Chase, S. B., editor, Problems in Public Expendi-
ture Analysis, pages 127–162. Brookings Institution,
Washington, DC.
Selinger, E., Neuenschwander, M., Koller, A., Gojda, J.,
Kuehn, T., Schwingshackl, L., Barbaresko, J., and
Schlesinger, S. (2023). Evidence of a vegan diet for
health benefits and risks–an umbrella review of meta-
analyses of observational and clinical studies. Critical
reviews in food science and nutrition, 63(29):9926–
9936.
Seto, K. C., G
¨
uneralp, B., and Hutyra, L. R. (2012). Global
forecasts of urban expansion to 2030 and direct im-
pacts on biodiversity and carbon pools. Proceedings
of the National Academy of Sciences, 109(40):16083–
16088.
Shaw, P., Price, R., Elliott, K., Copilah-Ali, J., Huntingford,
J., and Ionescu, O. (2024). White paper-corporate dig-
ital responsibility (cdr) securing our digital futures.
Corporate Digital Responsibility (CDR) Securing Our
Digital Futures, pages 1–35.
Singer, P. (1990). The significance of animal suffering. Be-
havioral and Brain Sciences, 13(1):9–12.
Stroia, N., Moga, D., Muresan, V., and Lodin, A. (2020).
Estimating environmental variables in smart sensor
networks with faulty nodes. In SMARTGREENS,
pages 67–73.
Vergallo, R., Cagnazzo, A., Mele, E., and Casciaro, S.
(2024a). Measuring the effectiveness of the ‘batch op-
erations’ energy design pattern to mitigate the carbon
footprint of communication peripherals on mobile de-
vices. Sensors, 24(22):7246.
Vergallo, R., Errico, A., and Mainetti, L. (2024b). On the ef-
fectiveness of the’follow-the-sun’strategy in mitigat-
ing the carbon footprint of ai in cloud instances. Avail-
able at SSRN 4566638.
Vergallo, R. and Mainetti, L. (2024). Measuring the ef-
fectiveness of carbon-aware ai training strategies in
cloud instances: A confirmation study. Future Inter-
net, 16(9):334.
Viscusi, W. K. (1993). The value of risks to life and health.
Journal of Economic Literature, 31(4):1912–1946.
Viscusi, W. K. (2019). Value of a statistical life. Oxford
Research Encyclopedia of Economics and Finance.
Wallace, P., Martin, R., and White, I. (2018). Keeping pace
with technology: Drones, disturbance and policy defi-
ciency. Journal of Environmental Planning and Man-
agement, 61(7):1271–1288.
SMARTGREENS 2025 - 14th International Conference on Smart Cities and Green ICT Systems
154