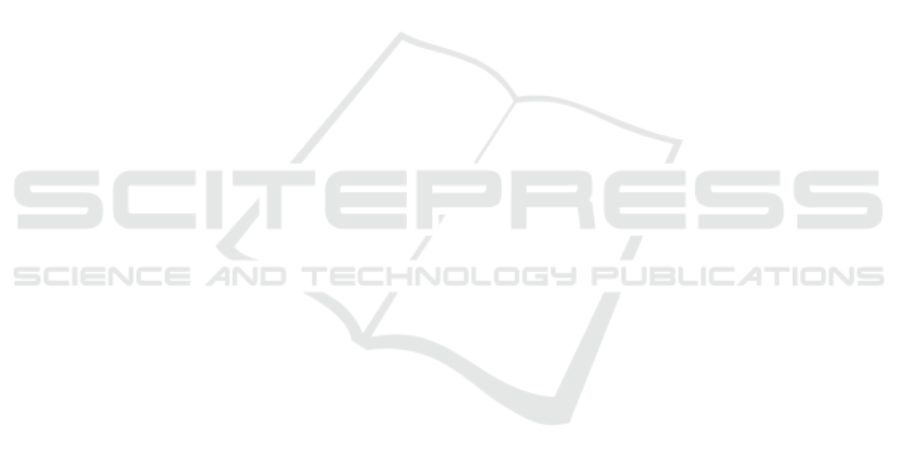
5 CONCLUSIONS
We test the feasibility of using a low-cost GNSS
receiver and antenna for land surveying based on
corrections broadcast from a collaborative network of
RTK mount points in France. The interface is SW
Maps, a free GIS Android application for
smartphones. We conclude that the nearest centipede
mount point does not mean that it is the best one to
use. The indicators of accuracy are not representative
in real-time using the SW Map smartphone
application and the mount points of the collaborative
free RTK network (Centipede). The corrections sent
by Centipede mount points may change dramatically
without any warning message for users in real-time.
The use of low-cost GNSS receivers/antennas with
the Centipede-RTK network connected via SW Maps
GIS free smartphone application should be controlled
and verified to ensure the reliability of the corrections
used for land surveying.
For Future work, we intend to automatically
record the data without the user intervention in the
SW Map to generate temporal series for long-term
measurement. Test the differences near ENSG,
ENSG2, LAUR, and OUIL mount points within less
than 10 km to examine the results of our work. Use
the corrections of Centipede using a high-quality
receiver-antenna and compare at the same time with
low-cost GNSS receiver-antenna results for the same
conditions to examine if there are notable differences.
Explore the possibility of using Centipede corrections
as an alternative solution in RTK mode for high-
quality receivers when the paid network broadcast is
lost. Finally, explore the archive of Centipede RTK
databases in RINEX format newly available in the
RENAG databases.
REFERENCES
Arrêté du 16 septembre 2003 portant sur les classes de
précision applicables aux catégories de travaux
topographiques réalisés par l’Etat, les collectivités
locales et leurs établissements publics ou exécutés pour
leur compte - Légifrance [WWW Document], n.d. URL
https://www.legifrance.gouv.fr/jorf/id/JORFTEXT000
000794936 (accessed 2.18.25).
Bellone, T., Dabove, P., Manzino, A.M., Taglioretti, C.,
2016. Real-time monitoring for fast deformations using
GNSS low-cost receivers. Geomatics, Natural Hazards
and Risk 7, 458–470.
https://doi.org/10.1080/19475705.2014.966867
Broekman, A., Gräbe, P.J., 2021. A low-cost, mobile real-
time kinematic geolocation service for engineering and
research applications. HardwareX 10, e00203.
https://doi.org/10.1016/j.ohx.2021.e00203
Calculs GNSS Réseau en ligne | RGP [WWW Document],
n.d. URL https://rgp.ign.fr/SERVICES/calcul_online.
php (accessed 9.8.22).
Caldera, S., Realini, E., Barzaghi, R., Reguzzoni, M.,
Sansò, F., 2016. Experimental study on low-cost
satellite-based geodetic monitoring over short
baselines. Journal of Surveying Engineering 142,
04015016.
Calibrated Survey GNSS Tripleband + L-band antenna
(IP67) [WWW Document], n.d. Geo-matching. URL
https://geo-matching.com/products/calibrated-survey-
gnss-tripleband-l-band-antenna-ip67 (accessed
2.18.25).
Cina, A., Piras, M., 2015. Performance of low-cost GNSS
receiver for landslides monitoring: test and results.
Geomatics, Natural Hazards and Risk 6, 497–514.
https://doi.org/10.1080/19475705.2014.889046
GNSS Science Support Centre fosters collaboration across
scientific communities through the provision of GNSS
science-based products and services., 2021. GSSC
Home [WWW Document]. URL https://gssc.esa.int/
(accessed 2.19.25).
Hamza, V., Stopar, B., Ambrožič, T., Sterle, O., 2021a.
Performance Evaluation of Low-Cost Multi-Frequency
GNSS Receivers and Antennas for Displacement
Detection. Applied Sciences 11, 6666.
https://doi.org/10.3390/app11146666
Hamza, V., Stopar, B., Ambrožič, T., Turk, G., Sterle, O.,
2020. Testing Multi-Frequency Low-Cost GNSS
Receivers for Geodetic Monitoring Purposes. Sensors
20, 4375. https://doi.org/10.3390/s20164375
Hamza, V., Stopar, B., Sterle, O., 2021b. Testing the
Performance of Multi-Frequency Low-Cost GNSS
Receivers and Antennas. Sensors 21, 2029.
https://doi.org/10.3390/s21062029
Hamza, V., Stopar, B., Sterle, O., Pavlovčič-Prešeren, P.,
2023. Low-Cost Dual-Frequency GNSS Receivers and
Antennas for Surveying in Urban Areas. Sensors 23,
2861. https://doi.org/10.3390/s23052861
INRAE: research for Agriculture, Food and Environment
[WWW Document], n.d. URL https://www.inrae.fr/en
(accessed 2.18.25).
Jackson, J., Saborio, R., Anas Ghazanfar, S., Gebre-
Egziabher, D., Davis, B., University of Minnesota.
Department of Aerospace Engineering and Mechanics,
2018. Evaluation of Low-Cost, Centimeter-Level
Accuracy OEM GNSS Receivers (No. MN/RC 2018-
10).
Le Reseau Centipede RTK [WWW Document], n.d.
Centipede RTK. URL https://docs.centipede.fr/
(accessed 2.18.25).
Leica Viva GS10 et GS25 – Récepteurs GNSS de haute
précision [WWW Document], n.d. URL https://leica-
geosystems.com/fr-fr/products/gnss-
systems/receivers/leica-viva-gs10-gs25 (accessed
2.18.25).
Sammuneh, M.A., El Meouche, R., Eslahi, M., Farazdaghi,
E., 2023. Low-Cost Global Navigation Satellite System
Low-Cost GNSS Receivers Reliability Using Centipede RTK Network for Land Surveying
217