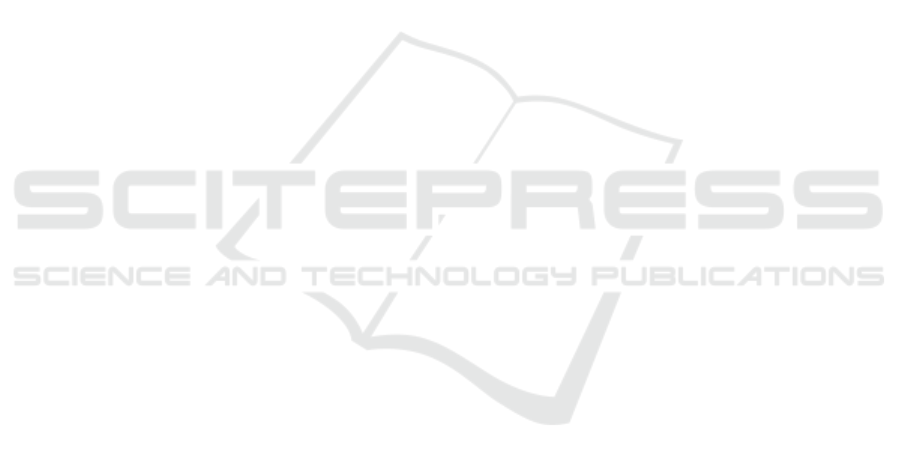
for both health and learning outcomes, reinforc-
ing the role of ergonomic training as a standard
component of surgical education.
6 CONCLUSION AND FUTURE
WORK
Minimally invasive pediatric surgery presents signifi-
cant ergonomic challenges that may increase the risk
of MSDs among surgeons. This study introduced a
real-time posture monitoring system that leverages
depth sensors and 3D skeletal tracking to provide
immediate feedback on postural deviations, with the
goal of enhancing both surgeon well-being and pro-
cedural efficiency. The system ensures privacy pro-
tection and offers seamless, unobtrusive integration
into the operating room without disrupting workflow.
While the system shows promise, further validation
in routine surgical environments is necessary. Critical
aspects to assess include its stability in tracking the
surgeon despite occlusions from medical staff, accu-
racy under varying lighting conditions, and the poten-
tial for false alarms that could interfere with concen-
tration. Beyond surgical practice, this study also ex-
plored the integration of posture monitoring into sur-
gical education and training. Real-time feedback, per-
sonalized learning pathways, and gamification strate-
gies could enhance trainee engagement and encour-
age the early adoption of proper ergonomic habits.
Future research will focus on experimental valida-
tion and evaluating the system’s applicability across
different surgical specialties. Additionally, long-term
studies will examine the impact of integrating posture
monitoring technology into educational programs, as-
sessing its effects on ergonomic awareness, trainee
evaluation, and improvements in occupational health
outcomes.
REFERENCES
Alleblas, C. C., De Man, A. M., Van Den Haak, L., Vier-
hout, M. E., Jansen, F. W., and Nieboer, T. E. (2017).
Prevalence of musculoskeletal disorders among sur-
geons performing minimally invasive surgery: a sys-
tematic review. Annals of surgery, 266(6):905–920.
Antico, M., Balletti, N., Laudato, G., Lazich, A., Notaran-
tonio, M., Oliveto, R., Ricciardi, S., Scalabrino, S.,
and Simeone, J. (2021). Postural control assessment
via microsoft azure kinect dk: An evaluation study.
Computer Methods and Programs in Biomedicine,
209:106324.
Anwary, A. R., Cetinkaya, D., Vassallo, M., Bouchachia,
H., et al. (2021). Smart-cover: A real time sitting
posture monitoring system. Sensors and Actuators A:
Physical, 317:112451.
Ayvaz,
¨
O.,
¨
Ozyıldırım, B. A.,
˙
Is¸sever, H.,
¨
Oztan, G., Atak,
M., and
¨
Ozel, S. (2023). Ergonomic risk assessment
of working postures of nurses working in a medical
faculty hospital with reba and rula methods. Science
Progress, 106(4):00368504231216540.
Bertram, J., Kr
¨
uger, T., R
¨
ohling, H. M., Jelusic, A.,
Mansow-Model, S., Schniepp, R., Wuehr, M., and
Otte, K. (2023). Accuracy and repeatability of the mi-
crosoft azure kinect for clinical measurement of motor
function. PLoS One, 18(1):e0279697.
Bigdelou, A., Schwarz, L., and Navab, N. (2012). An adap-
tive solution for intra-operative gesture-based human-
machine interaction. In Proceedings of the 2012
ACM international conference on Intelligent User In-
terfaces, pages 75–84.
Chai, J. and Hodgins, J. K. (2007). Constraint-based mo-
tion optimization using a statistical dynamic model.
In ACM SIGGRAPH 2007 papers, pages 8–es. ACM.
Dabholkar, T., Dabholkar, Y. G., Yardi, S., and Sethi, J.
(2020). An objective ergonomic risk assessment of
surgeons in real time while performing endoscopic si-
nus surgery. Indian Journal of Otolaryngology and
Head & Neck Surgery, 72(3):342–349.
Flouty, E., Zisimopoulos, O., and Stoyanov, D. (2018).
Faceoff: Anonymizing videos in the operating rooms.
In Stoyanov, D., Taylor, Z., Sarikaya, D., McLeod,
J., Gonz
´
alez Ballester, M. A., Codella, N. C., Mar-
tel, A., Maier-Hein, L., Malpani, A., Zenati, M. A.,
De Ribaupierre, S., Xiongbiao, L., Collins, T., Re-
ichl, T., Drechsler, K., Erdt, M., Linguraru, M. G.,
Oyarzun Laura, C., Shekhar, R., Wesarg, S., Celebi,
M. E., Dana, K., and Halpern, A., editors, OR 2.0
Context-Aware Operating Theaters, Computer As-
sisted Robotic Endoscopy, Clinical Image-Based Pro-
cedures, and Skin Image Analysis, pages 30–38,
Cham. Springer International Publishing.
Gallo, L. (2013). A study on the degrees of freedom in
touchless interaction. In SIGGRAPH Asia 2013 Tech-
nical Briefs, pages 1–4. ACM.
Gallo, L., Placitelli, A. P., and Ciampi, M. (2011).
Controller-free exploration of medical image data:
experiencing the kinect. In 2011 24th Interna-
tional Symposium on Computer-Based Medical Sys-
tems (CBMS), page 1–6. IEEE.
Haidegger, T., Speidel, S., Stoyanov, D., and Satava,
R. M. (2022). Robot-assisted minimally invasive
surgery—surgical robotics in the data age. Proceed-
ings of the IEEE, 110(7):835–846.
Hu, H., Hachiuma, R., Saito, H., Takatsume, Y., and Ka-
jita, H. (2022). Multi-camera multi-person tracking
and re-identification in an operating room. Journal of
Imaging, 8(8):219.
Huang, W., Chen, J., Zhao, X., and Liu, Q. (2021). Per-
formance evaluation of azure kinect and kinect 2.0
and their applications in 3d key-points detection of
students in classroom environment. In International
Conference on Artificial Intelligence in Education
Technology, pages 177–193. Springer.
Jara, H. V., Orejuela, I. Z., and Baydal-Bertomeu, J. (2022).
Study of the ergonomic risk in operators of an assem-
Real-Time 3D Posture Tracking for Surgeons in Pediatric Minimally Invasive Surgery
927